- 1School of Pharmacy, and Research Institute of Integrated TCM and Western Medicine, Chengdu University of Traditional Chinese Medicine, Chengdu, China
- 2State Key Laboratory of Southwestern Chinese Medicine Resources, Innovative Institute of Chinese Medicine and Pharmacy, Chengdu University of Traditional Chinese Medicine, Chengdu, China
- 3Ethnic Medicine Academic Heritage Innovation Research Center, Chengdu University of Traditional Chinese Medicine, Chengdu, China
Background: Most Aconitum species in traditional Chinese medicine have the effect of dispelling wind, dehumidifying, warming the meridian, and relieving pain. Aconitine is the characteristic chemical component with the function of anti-inflammation, analgesic, and heart-strengthening effects. However, improper use will produce cardiotoxicity and neurotoxicity. Currently, the mechanisms of cardiotoxicity caused by aconitine are wheels within wheels without being fully disclosed. The systematic review and meta-analysis were therefore conducted to summarize the available evidence of myocardial toxicity caused by aconitine.
Methods: We searched PubMed, Embase, Web of Science, National Knowledge Infrastructure, WANFANG, and VIP information database for relevant preclinical studies. All the data were analyzed by RevMan version 5.3.
Results: Thirty-two studies met the final inclusion criteria, including both in vivo and in vitro study types. After aconitine treatment, the heart rate of animals was obviously abnormal, and the morphology and function of myocardial cells were significantly changed. Aconitine can induce changes in the electrophysiological activity of cardiac myocytes by regulating Na+, Ca2+, and K+ currents. Meanwhile, the mechanisms of cardiotoxicity of aconitine may be related to triggering mitochondrial dysfunction by inducing mitochondrial apoptosis and autophagy. It should not be ignored that the overactivation of NLRP3 inflammasome also exacerbates aconitine’s cardiotoxicity.
Conclusion: The altered ion channels and mitochondrial function, as well as the signaling pathways interacting with NLRP3, may deserve further study for aconitine-induced cardiotoxicity.
Introduction
As common clinical traditional Chinese medicine in China, the main pharmacological effects of Aconitum species are cardiotonic, antihypertensive, anti-inflammatory, analgesic, and anti-tumor (Zhou et al., 2015), is highly regarded by physicians and pharmacists of various dynasties. According to historical documents and modern clinical reports, there are more than 600 prescriptions containing Aconitum species (Singhuber et al., 2009). Aconitine is a C19-diester diterpenoid alkaloid extracted from Aconitum species (Zhou et al., 2021), for example, Aconitum flavum Hand.-Mazz., Aconitum kusnezoffii Rchb., and Aconitum tschangbaischanense S. H. Li et Y. H. Huang (Figure 1). As the key substance that produces pharmacologic and toxicological effects, it has been widely reported that improper use of aconitine will cause toxicity to the cardiovascular system and central nervous system (Chan et al., 1993). By reviewing the previous literature, a total of 5,000 cases of aconitine poisoning were announced worldwide between 2001 and 2010, among which adverse cardiac events were the most serious clinical features (Li et al., 2016; Zhou et al., 2021). Therefore, the heart is the key target organ of aconitine toxicity. As reported, polymorphic ventricular arrhythmias, including the induction of ventricular premature beats (VPBs), atrioventricular blockade (AVB), ventricular tachycardia (VT), and ventricular fibrillation (VF), are the most common cardiac toxic side effects of aconitine (Lin et al., 2004; Ye et al., 2021; Zhou et al., 2021). Unfortunately, there is no effective antidote for aconitine-induced cardiotoxic events, which severely restricts the clinical use of aconitine-related traditional Chinese medicine or ethnic medicine. At present, the mechanism of aconitine-induced cardiotoxicity is not completely clear and needs further study. Hence, a comprehensive understanding of the molecular mechanism of aconitine cardiotoxicity will contribute to expanding the scope of clinical use of Aconitum medicinal materials.
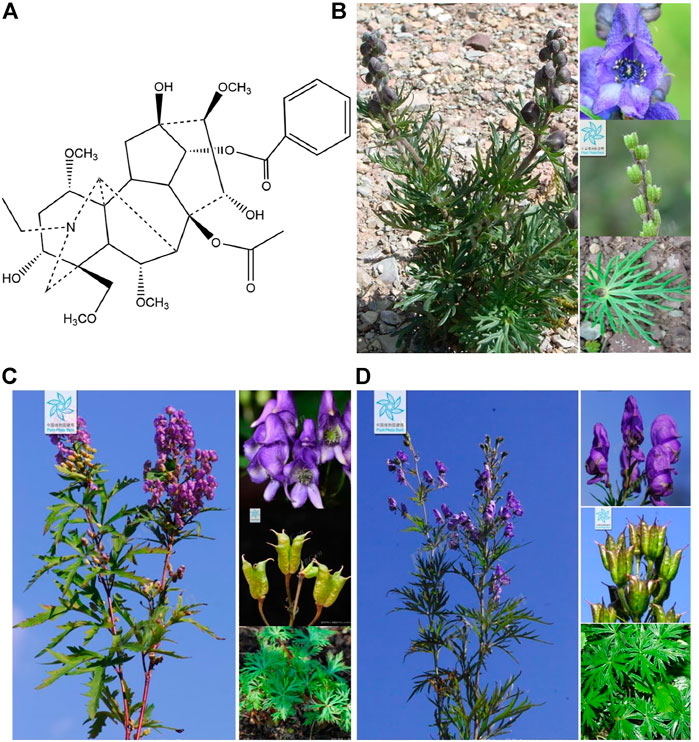
FIGURE 1. (A) Chemical structural formula of aconitine, (B) Aconitum flavum Hand.-Mazz., (C) Aconitum kusnezoffii Rchb., and (D) Aconitum tschangbaischanense S. H. Li et Y. H. Huang. The representative photographs of three Aconitum species were obtained from the Chinese flora online website (http://ppbc.iplant.cn/).
In this study, the mechanism of aconitine induced cardiotoxicity was reviewed from the following aspects: 1) the interaction between ion channels leads to intracellular overload of Na+ and Ca2+ and induces arrhythmia (Ching et al., 2000; Zhou et al., 2005); 2) the mitochondrial damage leads to cellular energy metabolism disorder by balancing the redox signaling pathway during the process of energy metabolism (Sun et al., 2014); 3) the overexpression of apoptosis and autophagy-related proteins; 4) the activation of NLRP3 signaling pathway and its downstream caspase-1, IL-18, and IL-1β, leading to cardiac injury (Kang and Leaf, 1996; Yamamoto et al., 2000; Xiong et al., 2006; Gao et al., 2018). Meanwhile, we also give effective measures to reduce the cardiotoxicity of aconitine.
Systematic review is a powerful means of providing reliable information, which could be considered to be of the uppermost level of medical evidence. Based on the level of evidence from the Centre of Evidence-Based Medicine in Oxford, only data from a systematic review would be proposed as 1a-evidence (Glasziou et al., 2004). As an effective and widely accepted method, the review of a large amount of preclinical evidence of aconitine cardiotoxicity in vivo and in vitro will help to expand its clinical application and to deal with sudden poisoning events. Therefore, our goal is to determine the mechanism of aconitine cardiotoxicity by a comprehensive systematic review and meta-analysis.
Methods
Search Strategy
We used the public online databases of PubMed, Embase, Web of Science, National Knowledge Infrastructure (CNKI), WANFANG, and the VIP information to perform a comprehensive retrieval. Also then, we analyzed the in vivo and in vitro mechanism of aconitine-induced cardiotoxicity. From January 1980 to November 2021, all searches were documented electronically. The following was our technique for conducting a literature search: (aconitine OR aconite alkaloid OR aconitine alkaloids) AND (cardiotoxicity OR cardiac toxicity OR myocardial toxicity OR myocardial damage OR cardiovascular toxicity OR heart toxic OR heart toxicity).
Study Selection
Experimental investigations evaluating the mechanisms of aconitine-induced cardiotoxicity were selected and incorporated. According to the above retrieval strategies, two authors were separately delegated to review the titles and/or abstracts, and then assess the qualifications of the full-text articles. The following criteria were used to determine whether or not a study should be included: 1) the article about aconitine causes cardiotoxicity; 2) the experimental group received aconitine monotherapy intervention despite mode, dosage, and frequency; 3) the major outcomes examined were the heart and myocardial cell injury (including at least one relevant indicator); 4) the control group received normal saline or no adjunct intervention. Correspondingly, the following were the predetermined exclusion criteria: 1) cardiotoxicity was not the target ailment; 2) aconitine was used in combination; 3) the article was a clinical study; 4) the study was a case report, clinical trial, review, abstract, comment, conference paper, or duplicate publication; and 5) no control group was included.
Extraction of Information
The articles were evaluated by two independent reviewers, and the following particulars were retrieved from chosen studies: 1) the first author and the year of publication; 2) individual data from each study, such as animal species, gender, samples for individual comparison and weight; 3) type of anesthetic; 4) characteristics of intervention in treatment and control groups, such as drug, dosage, method of treatment, and frequency; 5) mean value, standard deviation, and inter-group difference of measurement and the corresponding data. If the presented data was incomplete and ambiguous, we attempted to contact the authors for more information or using digital ruler software else we just performed a qualitative analysis.
Quality Evaluation
Two authors independently evaluated the methodological quality of the included studies based on the list of collaborative approach to meta-analysis and review of animal data from experimental studies (CAMARADES). The 10-item checklist was as follows (Sena et al., 2007): 1) peer-reviewed publication; 2) statements of temperature control; 3) randomly divided into treatment group or control group; 4) the model was induced by blind method; 5) blinded evaluation of results; 6) use of anesthetic had no apparent intrinsic myocardial preservation or neuroprotective effect; 7) use of animals with relevant comorbidities; 8) sample size calculation; 9) obedience with animal welfare regulations, and 10) declared any underlying conflict of benefits. Each research received an overall quality rating ground on a one-point system for each item. For any article we have questions about, we will immediately contact the corresponding author to discuss and negotiate.
Statistical Analysis
RevMan version 5.3 software was employed to perform the pooled analyses. All of the outcome variables were treated as continuous data. The random effects model and standard mean difference (SMD) with 95% confidence intervals (CIs) were used to evaluate the mechanism of aconitine-induced cardiotoxicity. The I2 statistics test was used to examine heterogeneity among individual research. If the probability value was <0.05, the difference was considered statistically significant.
Results
Study Inclusion
Following a rigorous search of six databases, we found 6,461 publications with 6,207 records remaining after duplicates. Also, 6,148 publications were ruled out based on their titles and abstracts for at least one of the following reasons: 1) review article, commentary, conference article, or clinical study; 2) with no interesting outcomes; 3) other reasons, such as a letter to the editor and books. After scanning the complete text of the remaining 59 studies, six studies were eliminated because they are duplicate publications (such as dissertations and articles). Moreover, 21 studies were deleted because the outcome measurement was not of interest. Ultimately, 32 eligible articles (Liang et al., 1991; Meng, 2006; Fu, 2007; Liu, 2007; Wang, 2007; Wang et al., 2007; Zhang, 2007; Xu, 2008; Liu, 2009; Deng, 2010; Fang et al., 2012; Zhang et al., 2012; Wang, 2013; Zhou et al., 2013; Sun et al., 2014; Yu, 2015; Cui et al., 2018; Gao, 2018; Gao et al., 2018; Hu et al., 2018; Li et al., 2018; Zhang et al., 2018; Liu et al., 2019; Wang M. et al., 2020; Li et al., 2020; Peng et al., 2020; Zhang et al., 2020; Zhou et al., 2020; Xia et al., 2021; Yang et al., 2021; Ye et al., 2021; Wang S. et al., 2022) were identified for further evaluation, data extraction, and analysis (Figure 2).
Characteristics of Included Studies
Table 1 summarized the essential characteristics of thirty-two qualifying research, between 1991 and 2021, with 12 studies done in English and 20 research conducted in Chinese. For study type and animal species, 27 studies were in vitro experiments, three studies were in vivo experiments, and two studies (Wang, 2013; Sun et al., 2014) had both in vitro and in vivo experiments. Of them, the animals used in three studies (Liang et al., 1991; Zhang et al., 2012; Sun et al., 2014) comprised Sprague–Dawley (SD) and Wistar rats, and two studies (Deng, 2010; Wang, 2013) guinea pigs. The weight of rats ranged from 150 to 350 g, and the weight of guinea pigs ranged from 300 to 350 g. Among which, zebrafish embryos were employed in seven studies (Fang et al., 2012; Cui et al., 2018; Liu et al., 2019; Wang S. et al., 2020; Li et al., 2020; Xia et al., 2021; Ye et al., 2021), H9c2 cell in nine studies (Gao, 2018; Gao et al., 2018; Hu et al., 2018; Zhang et al., 2018; Li et al., 2020; Peng et al., 2020; Zhang et al., 2020; Yang et al., 2021; Wang W. et al., 2022), primary cardiomyocytes of SD rats in 10 studies (Meng, 2006; Fu, 2007; Wang, 2007; Wang et al., 2007; Zhang, 2007; Xu, 2008; Liu, 2009; Zhou et al., 2013; Yu, 2015; Li et al., 2018), primary cardiomyocytes of Wistar rats in three studies (Liu, 2007; Sun et al., 2014; Zhou et al., 2020). Meanwhile, three studies chose primary cardiomyocytes of guinea pigs (Wang, 2013) and AC-16 cells (Cui et al., 2018; Zhou et al., 2020). For the choice of anesthesia in animal experiments, two studies (Zhang et al., 2012; Yu, 2015) used chloral hydrate, one study (Sun et al., 2014) ketamine/xylazine, and one study (Liu et al., 2019) MS-222, three studies (Wang, 2013; Zhou et al., 2013; Sun et al., 2014) pentobarbital, and the rest of studies was not reported. Of the 32 included studies, each one had different measured indicators, as well as different routes, times, and dosages of administration. In the results column, the arrow symbols pointing in different directions were used to indicate the trends of each indicator. The detailed information was summarized in Table 1.
Cardiotoxic Mechanisms
Cell Viability
When a toxic substance acts on mammalian cells, the most obvious manifestation is a decrease in cell vitality. Therefore, cell viability is often used as target organ toxicity for rapid and mass screening of natural products. In the present study, fifteen articles measured the index of cell viability, among which ten articles revealed that aconitine signally inhibited cardiomyocyte activity in a dose-dependent manner. Comparatively, there was evidence from one article that aconitine (5–1,280 μM) had no obvious effect on myocardial cell viability compared to the control group (Liu, 2007). A little regrettably, two articles did not carry out statistical analysis (Xu, 2008; Zhou et al., 2020). The data integrity analysis from two articles manifested a noticeable decline in myocardial cell viability by aconitine administration [n = 40, SMD = −1.19, 95% CI (−2.05 to −0.33), p = 0.007; heterogeneity: χ2 = 22.21, df = 1 (p < 0.00001), I2 = 95%] (Figure 3A) (Wang et al., 2007; Hu et al., 2018).
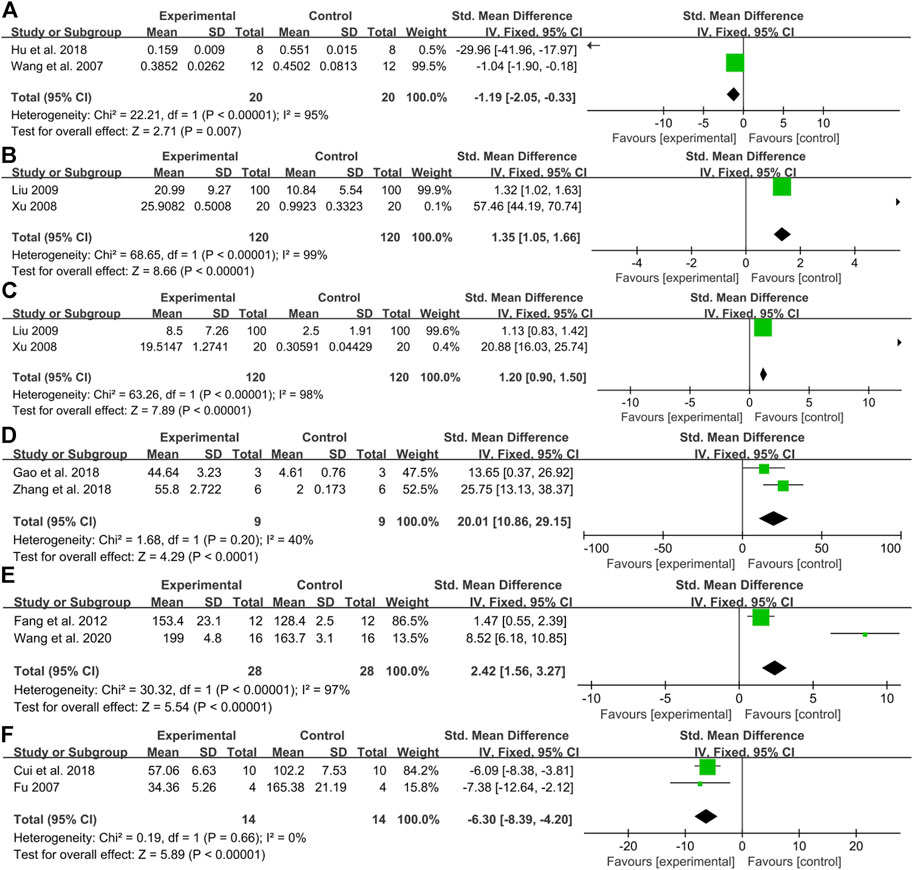
FIGURE 3. Pooled estimate of DNA damage: (A) cell viability, (B)TDNA%, (C) TM, (D) apoptosis rate, (E) heart rate, and (F) Ca2+ transient amplitude.
DNA Damage
As the main signal of cell apoptosis, the serious DNA damage cannot be repaired, resulting in programmed death. In our results, three studies investigated the effect of aconitine in DNA damage, including HDNA%, TDNA%, TM, TL, and OTM (Fu, 2007; Xu, 2008; Liu, 2009). It is found that HDNA% decreased gradually, while TDNA%, TM, TL, and OTM increased gradually after aconitine administration with concentration dependency. The meta-analysis of two studies (Xu, 2008; Liu, 2009) showed a significant increase in TDNA% [n = 240, SMD = 1.35, 95% CI (1.05 to 1.66), p < 0.00001; heterogeneity χ2 = 68.65, df = 1 (p < 0.00001), I2 = 99%] (Figure 3B), and a significant increase in TM [n = 240, SMD = 1.20, 95% CI (0.90 to 1.50), p < 0.00001; heterogeneity χ2 = 63.26, df = 1 (p < 0.00001), I2 = 98%] (Figure 3C).
Apoptosis Rate
A large number of cardiomyocyte apoptosis is also considered a crucial marker for evaluating the cardiotoxicity of compounds (Hu et al., 2018). In our study, the apoptosis rate was conducted in seven studies (Gao, 2018; Hu et al., 2018; Zhang et al., 2018; Liu et al., 2019; Li et al., 2020; Xia et al., 2021; Yang et al., 2021). The meta-analysis of two studies (Gao, 2018; Zhang et al., 2018) showed that compared with the control group, the apoptosis rate prominently surged and boomed in a concentration-dependent manner [n = 18, SMD = 20.01, 95% CI (10.86 to 29.15), p < 0.0001; heterogeneity χ2 = 1.68, df = 1 (p = 0.20), I2 = 40%] (Figure 3D).
Heart Rate
Most drugs that act on the cardiovascular system are known to affect the heart rate to some extent. A heart rhythm that is too fast or too slow is not conducive to maintaining normal heart function. Similarly, heart rate has been used as an indispensable and flexible indicator to evaluate the cardiotoxicity of compounds (Ye et al., 2021). It was mentioned in five studies (Fu, 2007; Wang et al., 2007; Liu, 2009; Sun et al., 2014; Zhou et al., 2020) that after aconitine was applied to animals, the beating frequency of the heart was first increased, and then, the state similar to convulsion appeared. With the passage of time, there was a gradual decline in the beating frequency, and the rhythm become even worse and more random. The heart rate of SD rats was seen in clear rising in one study (Zhang et al., 2012). Conformably, aconitine-evoked a remarkable rise in heart rate was also demonstrated in zebrafish embryo models compared with the control group in a dose-response relationship (Fang et al., 2012; Cui et al., 2018; Wang M. et al., 2020; Xia et al., 2021; Ye et al., 2021). A meta-analysis of two studies (Fang et al., 2012; Wang S. et al., 2020) for the increased heart rate in zebrafish embryos [n = 56, SMD = 2.42, 95% CI (1.56 to 3.27), p < 0.00001; heterogeneity: χ2 = 30.32, df = 1 (p < 0.00001), I2 = 97%] (Figure 3E).
Ca2+ Transients
There is considerable evidence that intracellular Ca2+ surge can lead to irreversible damage to cardiomyocytes through interaction with oxidative stress and the proliferation of reactive oxygen species (ROS), which can eventually trigger an apoptotic cascade of cardiomyocytes or further exacerbation of pre-existing heart disease. The Ca2+ modulators are therefore widely used to screen drugs for the cardiovascular system (Fu et al., 2008). Compared with the controls, six studies concluded that the administration of aconitine could increase Ca2+ oscillation frequency, while decreasing its oscillation amplitude resulting in cardiotoxicity via overloaded intracellular Ca2+ and changed cardiac electrophysiological characteristics in a concentration-dependent manner (Fu, 2007; Zhou et al., 2013; Sun et al., 2014; Yu, 2015; Cui et al., 2018; Li et al., 2018). The meta-analysis of two studies indicated that aconitine treatment repressed the oscillation amplitude of Ca2+ [n = 28, SMD = −6.03, 95% CI (−8.39 to −4.20), p < 0.00001; heterogeneity: χ2 = 0.19, df = 1 (p = 0.66), I2 = 0%] (Figure 3F) (Fu, 2007; Cui et al., 2018).
So to sum it up, the main mechanisms of aconitine-induced cardiotoxicity comprised impaired cell viability, ineluctable DNA damage, excessive apoptosis, abnormal heart rate, and overloaded intracellular Ca2+ concentration. When the value of I2 is greater than 50%, subgroup analysis is needed to explore the potential source of high heterogeneity. However, many included studies lack quantitative data for integration and multi-dimensional analysis, leading to the large heterogeneity in reasonably and appropriately evaluating the potential mechanisms of aconitine-induced cardiotoxicity. The future in vitro and in vivo experiments with larger samples should be urgently performed to elucidate its multifaceted molecular mechanisms on cardiotoxicity.
Study Quality
Through a holistic and comprehensive evaluation of the included literature, 13 studies had a quality assessment range of 2/10 to 9/10 with an average of 5.92 in Table 2. Of which, one study (Sun et al., 2014) got nine points, one study (Peng et al., 2020) got eight points and four studies (Wang M. et al., 2020; Li et al., 2020; Xia et al., 2021; Ye et al., 2021) got seven points, two studies (Cui et al., 2018; Liu et al., 2019) got six points, two studies (Fang et al., 2012; Wang, 2013) got five points, two studies (Liang et al., 1991; Zhang et al., 2012) got four points, and the rest of the study (Deng, 2010) got two points. All studies were published in peer-reviewed journals. Nine studies (Fang et al., 2012; Sun et al., 2014; Cui et al., 2018; Liu et al., 2019; Wang S. et al., 2020; Li et al., 2020; Peng et al., 2020; Xia et al., 2021; Ye et al., 2021) described control of the temperature. Eight studies (Liang et al., 1991; Fang et al., 2012; Zhang et al., 2012; Wang, 2013; Sun et al., 2014; Cui et al., 2018; Peng et al., 2020; Xia et al., 2021) were randomly assigned to treatment or control groups. Six studies (Sun et al., 2014; Cui et al., 2018; Wang M. et al., 2020; Li et al., 2020; Xia et al., 2021; Ye et al., 2021) described allocation concealment. All studies declared that the outcome assessment was blinded. The anesthetics used in the four studies (Wang, 2013; Sun et al., 2014; Liu et al., 2019; Peng et al., 2020) had no apparent intrinsic vascular protection activity. No studies used animals with associated comorbidities. One study (Deng, 2010) did not calculate the sample size in the experiment. Seven studies (Sun et al., 2014; Liu et al., 2019; Wang S. et al., 2020; Li et al., 2020; Peng et al., 2020; Xia et al., 2021; Ye et al., 2021) referred to abide by animal welfare regulations. Also, five studies (Sun et al., 2014; Wang M. et al., 2020; Li et al., 2020; Peng et al., 2020; Ye et al., 2021) contained notes on potential conflict of interests.
Discussion
Limitations
Some limitations should be taken into account when we interpret the underlying mechanisms of aconitine on cardiotoxicity through a systematic and comprehensive meta-analysis. First of all, only Chinese and English articles are searched, which may result in selection bias due to the lack of studies published in other languages. Second, each article investigating the cardiotoxicity of aconitine uses different drug administration methods, routes, doses, and times, resulting in the difference in various detection indexes and the incomparability of results. Third, the quality scores of less than five points indicate a low quality of method in included studies. Furthermore, most studies have shortcomings in randomized allocation, concealed blindness, and sample size calculation, which are core criteria for study design. Moreover, the articles do not provide primary data, leading to the failure for us to illuminate the cardiotoxicity mechanisms of aconitine based on multiple indexes by meta-analysis. Therefore, the mechanisms of aconitine on cardiotoxicity outlined in our study at different levels should be rationally viewed and explained. Overall, in the follow-up protocols on the cardiotoxicity caused by aconitine, the researchers can use a nearly identical or equivalent dose range, frequency, and route in the experimental implementation phase, so as to conduct an in-depth analysis of the mechanism with available original data.
Implications
The cardiotoxicity induced by aconitine is an intricate process, involving various factors and have not been interpreted completely. Until now, the main mechanisms of cardiotoxicity studies are mainly focused on interactions with multifarious ion channels, induction of mitochondrial dysfunction, as well evoking apoptosis, and autophagy. The specific mechanism of aconitine inducing cardiotoxicity mentioned above can provide a variety of options for further exploration by scientists of the Aconitum. In the meanwhile, these are widely recognized indicators that should be focused on in the development and clinical application of Chinese patent medicines containing Aconitum medicinal plants (Figure 4).
Interactions With Ion Channels
Na+, Ca2+, and K+ channels exist widely on the surface of myocardial cell membrane and any abnormal currents generated by these ion channels will lead to cardiotoxicity. The disruption of intracellular ion homeostasis due to the interactions between aconitine and ion channels has been proverbially considered to be the key mechanism of aconitine-induced arrhythmia. Aconitine, as an agonist to bind to voltage-gated Na+ channels on the cell membrane, could cause abnormal activation of Na+ channels and memorably increase Na+ influx, leading to intracellular Na+ overload. A previous study manifested that aconitine could augment the peak of INa by irritating Na+ influx in a concentration-dependent manner (Wang et al., 2021). On the other hand, evidence also suggested that aconitine could sharply elevate the expression of calmodulin ryanodine receptor 2 (RyR2) and Na+-Ca2+ exchanger (NCX), which may be the main culprit of its cardiotoxicity. The RyR2 channel has been proclaimed to induce the repetitive and persistent release of Ca2+ from the sarcoplasmic reticulum (SR), and thereupon enter the cytoplasmic matrix through the NCX, resulting in an explosion and overload of Ca2+ in the cytosol of the cardiomyocytes (Fu et al., 2007; Fu et al., 2008). Although aconitine could attenuate Ca2+ oscillations amplitude, the increased frequency of Ca2+ release from the SR ultimately contributes to the upregulation in cytoplasmic Ca2+ (Fu, 2007). The Ca2+ oscillations with higher frequencies substantially dephosphorylate the gap junction protein Cx43 at the Ser368 site resulting in changes in electrophysiological characteristics of cardiomyocytes (Liu, 2009). In general, intracellular Na+-Ca2+ overload is considered to be the basic pathogenesis of aconitine-induced arrhythmias.
Judging from the currently available evidence, generous studies have announced that aconitine-induced arrhythmia is related to K+ channel status. As a non-selective K+ channel blocker, aconitine can block transient outward of K+ current (Ito), ultra-rapid delayed rectifier K+ current (Ikur), and fast delay rectifier outward of K+ current (IKr). As a crucial Kv channel widely distributed in the heart, the aconitine-induced declines of Kv4.3 mRNA expression could change Ito current (Liu, 2007) and expressively prolong APD plateau, thus leading to arrhythmia by fluctuating cardiac electrophysiological frequency. Amazedly, a steady stream of evidence confirmed that aconitine can block the current of IKr, composed of α subunit HERG and β subunit MiRP1, by intercepting the HERG channel, causing the prolonged cardiac APD and arrhythmia (Abbott et al., 1999; Wang S. et al., 2020). After aconitine treatment on cardiomyocytes, it will accommodate the expressions of ion channel-related proteins, and eventually mediate the transposition of intracellular Na+, Ca2+, and K+, ultimately causing arrhythmia.
Mitochondrial Dysfunction
After aconitine administration, the pulsation of cardiomyocytes was reduced or even disappeared with the characteristics of a shrunken cell and smaller nucleus. The results of the electron microscope showed that aconitine could induce cavities in the cytoplasm, rough endoplasmic reticulum expansion, mitochondrial swelling, and rupture, as well crest fracture. In addition to changes in mitochondrial morphology, aconitine also perturbed mitochondrial energy metabolism, evidenced by the restrained activities of reductive nicotinamide adenine dinucleotide dehydrogenase (NADHD) (Liang et al., 1991), succinate dehydrogenase (SDH), and cytochrome oxidase (CCO), while the aggrandized lactate dehydrogenase (LDH) level (Meng, 2006; Fu, 2007; Liu, 2007; Liu, 2009; Sun et al., 2014; Zhang et al., 2018; Wang S. et al., 2022). As a consequence, the inhibited oxidative phosphorylation of the tricarboxylic acid cycle tremendously boycotted and intimidated ATP synthesis (Gao et al., 2018), resulting in the impaired energy metabolism of cardiomyocytes. Meanwhile aconitine downregulated the expression of PGC-1α (Gao et al., 2018), which efficaciously impeded mitochondrial biosynthesis and ATP synthesis. In addition to ATP production and yield, mitochondrial oxidative stress signaling had also been implicated in the cardiotoxicity of aconitine, such as mitochondrial reactive oxygen species (mtROS) and 8-hydroxy-2 deoxyguanosine (8-OHDG) oxidative damage indicators. Once the cardiomyocyte’s redox state was broken up by aconitine, massive mtROS and 8-OHDG would accumulate in the cytoplasm, (Xu, 2008; Gao, 2018; Wang S. et al., 2020; Peng et al., 2020; Xia et al., 2021; Wang W. et al., 2022), further exacerbating the mitochondrial structure and function disorder. In retrospect, we reconsidered that mitochondrial energy metabolism and oxidative stress damage may be nonnegligible and momentous molecular mechanisms of aconitine-evoked cardiotoxicity.
Apoptosis and Autophagy
Autophagy is a process that in which a mammalian cell engulfs its own cytoplasmic proteins or organelles and wraps them into vesicles. By fusing with lysosomes to form autophagic lysosomes, the pernicious cellular wastes and products are promptly removed and cleaned up (Wang et al., 2019b). As another cell death event that determines the fate of cells, apoptosis refers to the spontaneous and orderly cell death process under the regulation of apoptosis-related genes (Wang et al., 2019a). It was reported that the expression of autophagy proteins LC3 and Beclin-1, and pro-apoptotic associated proteins caspase-3/8/9 were markedly upregulated after aconitine treatment. Simultaneously, the expression of anti-apoptotic protein Bcl-2 is visibly downregulated (Sun et al., 2014; Hu et al., 2018; Peng et al., 2020; Wang S. et al., 2022). Therefore, maintaining the dynamic balance between autophagy and apoptosis may be an effective measure to relieve and rescue the cardiotoxicity of aconitine-related traditional Chinese medicine products.
NLRP3 Signaling Pathway
To date, the pathway involved in aconitine-induced cardiotoxicity has also been further demonstrated experimentally. Aconitine could miraculously activate the NLRP3 signaling pathway by abnormally increasing palmitic acid levels in cardiac tissue. Conversely, aconitine treatment or silencing of the NLRP3 gene can signally confine the expressions of caspase-1, IL-18, and IL-1β (Bi et al., 2020; Peng et al., 2020). From the available evidence, excessive NLRP3 inflammasome activation may be a potential signaling pathway for aconitine-induced cardiotoxicity. However, considering NLRP3’s pivotal role in determining cell fate, its mediated oxidative stress, mitochondrial energy metabolism, as well autophagy, and apoptosis events are consequential and meaningful targets for further exploration of aconitine-induced cardiotoxicity (Wang W. et al., 2022).
Measures to Reduce Cardiotoxicity of Aconitine
In recent years, cardiotoxic adverse events of aconite alkaloids emerge one after another, and its inevitable cardiotoxicity has critically limited the clinical use of Aconitum species (Zhou et al., 2021). Therefore, we have to take some effective and immediate measures to reduce its cardiotoxicity, so that it can be better used to treat a variety of clinical diseases. First, the cardiomyocyte toxicity of aconitine can be partly reduced through reasonable compatibility with some monomers, possibly via competitive occupation of toxic protein targets (Fei et al., 2015; Dong et al., 2017). Second, the toxicity of aconitine can be effectively reduced by decocting and/or boiling Aconitum medicinal herbs at a reasonable time and temperature. In this process, the tempestuously toxic diester-diterpenoid alkaloids are unstable and can be easily hydrolyzed into relatively less toxic monoester-diterpenoid alkaloids, and non-toxic non-esterified diterpene alkaloids (Li et al., 2022). In detail, aconitine can be hydrolyzed into benzoylaconine by removal of an acetyl group at the C-8 site via vanishing one molecule of acetic acid, followed by obtaining aconine by removal of a benzoyl group at the C-14 site via vanishing one molecule of benzoic acid at 100°C. Third, it has been widely accepted and approved that the orderly preparing process with other Chinese herbs, such as Hezi (Terminalia chebula Retz.) decoction, can miraculously reduce aconitine levels in Aconitum herbals (Li et al., 2022). For instance, the cardiotoxicity of Caowu (Aconiti Kusnezoffii Radix) was almost counteracted by soaking with Hezi decoction, evidenced by lessened ROS, LDH, and release of Ca2+, the increased mitochondrial membrane potential, the improved nuclear morphology, and the activity of Na+-K+-ATPase in H9c2 cells (Zhang et al., 2017; Han et al., 2022). In general, the cardiotoxicity caused by aconitine can be mitigated by optimal monomer compatibility, heat processing, and soaked with potential medicinal herbal materials. In addition, appropriate dosing and administration, such as local administration, are bound to reduce the cardiotoxicity of aconitine. Hopefully, the development of sustained release dosage forms of aconitine or targeted delivery systems for specific tissues and organs may be robust measures to reduce its cardiotoxicity.
Conclusion
As a violent poison, aconitine-induced cardiotoxicity was associated with changed electrophysiological characteristics of cardiomyocytes through interactions with Na+, Ca2+, and K+ channels, the dysfunction of the mitochondrion, the induction of apoptosis and autophagy, as well the activation of NLRP3 related signaling pathways. Although there are many effective methods such as monomer compatibility and diversified and distinctive processing methods of traditional Chinese medicine that reduce the cardiotoxicity of aconitine still a great deal of in vivo and in vitro experiments, as well as clinical trials are urgently needed to elucidate the dynamic processes of aconitine in animals and humans. Simultaneously, in-depth investigations into the toxic mechanisms of the heart and undiscovered potential organotoxicity still need to be further probed with the help of three-dimensional microfluidic organoid models.
Data Availability Statement
The original contributions presented in the study are included in the article/Supplementary Material; further inquiries can be directed to the corresponding authors.
Author Contributions
HJ designed the structure and drafted the manuscript. YZ and XW contributed to data extraction and interpretation. YZ, XW, and XM reviewed and revised the manuscript and finally approved the publication.
Funding
We are grateful for the financial support from the National Natural Science Foundation of China (82130113), the Regional Innovation and Cooperation Project of the Science and Technology Department of Sichuan Province (2020YFQ0032), and the Key R&D and Transformation Program of the Science and Technology Department of Qinghai Province (2020-SF-C33).
Conflict of Interest
The authors declare that the research was conducted in the absence of any commercial or financial relationships that could be construed as a potential conflict of interest.
Publisher’s Note
All claims expressed in this article are solely those of the authors and do not necessarily represent those of their affiliated organizations, or those of the publisher, the editors, and the reviewers. Any product that may be evaluated in this article, or claim that may be made by its manufacturer, is not guaranteed or endorsed by the publisher.
References
Abbott, G. W., Sesti, F., Splawski, I., Buck, M. E., Lehmann, M. H., Timothy, K. W., et al. (1999). MiRP1 Forms IKr Potassium Channels with HERG and Is Associated with Cardiac Arrhythmia. Cell. 97, 175–187. doi:10.1016/s0092-8674(00)80728-x
Bi, C., Zhang, T., Li, Y., Zhao, H., Zhang, P., Wang, Y., et al. (2020). A Proteomics- and Metabolomics-Based Study Revealed that Disorder of Palmitic Acid Metabolism by Aconitine Induces Cardiac Injury. Chem. Res. Toxicol. 33, 3031–3040. doi:10.1021/acs.chemrestox.0c00372
Chan, T. Y., Chan, J. C., Tomlinson, B., and Critchley, J. A. (1993). Chinese Herbal Medicines Revisited: a Hong Kong Perspective. Lancet 342, 1532–1534. doi:10.1016/s0140-6736(05)80091-1
Ching, L. L., Williams, A. J., and Sitsapesan, R. (2000). Evidence for Ca(2+) Activation and Inactivation Sites on the Luminal Side of the Cardiac Ryanodine Receptor Complex. Circ. Res. 87, 201–206. doi:10.1161/01.res.87.3.201
Cui, G., Li, T., Shi, W., Wang, L., and Li, M. (2018). Research on the Arrhythmia Model in Zebrafish and Human Cardiomyocytes-Induced by Aconitine. Chin. J. Clin. Pharmacol. 34, 1454–1456. doi:10.13699/j.cnki.1001-6821.2018.12.017
Deng, L. (2010). The Effect of Aconitine on the Action Potentials in Papillary Muscle and Sinoatrial of guinea Pig and its Underlying Mechanism. Beijing, China: Hebei University of Science & Technology. Master Thesis. CNKI online database. Available at: https://chn.oversea.cnki.net/index/.
Dong, Y., Xie, Y., Li, X., Zhu, Y., and Peng, C. (2017). Protective Effect of Compatibilities of Ginsenosides Rg1 and Aconitine on Myocardial Cells of In Vitro Cultured Heart Failure Model. China Pharm. 28, 472–475. doi:10.6039/j.issn.1001-0408.2017.04.11
Fang, F., Zhao, J., Yu, L., and Luo, J. (2012). Priliminary Investigation of Cardiac Toxicity to Zebrafish Embryo by Aconitine. Pharmacol. Clin. Chin. Materia Medica 28, 32–34. doi:10.13412/j.cnki.zyyl.2012.02.018
Fei, Q., Zhang, X., Dong, Y., Xie, X., and Peng, C. (2015). Study on Toxic Concentration of Aconitine Combined with Ginsenoside Rg1 on Cardiomyocytes. Lishizhen Med. Materia Medica Res. 26, 2610–2613. doi:10.3969/j.issn.1008-0805.2015.11.017
Fu, M., Li, R. X., Fan, L., He, G. W., Thornburg, K. L., and Wang, Z. (2008). Sarcoplasmic Reticulum Ca2+ Release Channel Ryanodine Receptor (RyR2) Plays a Crucial Role in Aconitine-Induced Arrhythmias. Biochem. Pharmacol. 75, 2147–2156. doi:10.1016/j.bcp.2008.02.027
Fu, M., Wu, M., Wang, J. F., Qiao, Y. J., and Wang, Z. (2007). Disruption of the Intracellular Ca2+ Homeostasis in the Cardiac Excitation-Contraction Coupling Is a Crucial Mechanism of Arrhythmic Toxicity in Aconitine-Induced Cardiomyocytes. Biochem. Biophys. Res. Commun. 354, 929–936. doi:10.1016/j.bbrc.2007.01.082
Fu, M. (2007). Molecular and Cellular Mechanism on Aconitine-Induced Arrhythmic Toxicity. Beijing, China: Beijing University of Chinese Medicine. Master Thesis. CNKI online database. Available at: https://chn.oversea.cnki.net/index/.
Gao, X., Zhang, X., Hu, J., Xu, X., Zuo, Y., Wang, Y., et al. (2018). Aconitine Induces Apoptosis in H9c2 Cardiac Cells via Mitochondria-mediated P-athway. Mol. Med. Rep. 17, 284–292. doi:10.3892/mmr.2017.7894
Gao, X. (2018). Aconitine Induces Apoptosis in H9c2 Cardiac Cells via Mitochondria-Mediated Pathway. Beijing, China: Soochow University. Master Thesis. CNKI online database. Available at: https://chn.oversea.cnki.net/index/.
Glasziou, P., Vandenbroucke, J. P., and Chalmers, I. (2004). Assessing the Quality of Research. BMJ 328, 39–41. doi:10.1136/bmj.328.7430.39
Han, S., Bao, L., Liu, K., Han, X., Tang, Y., Liu, Z., et al. (2022). Mechanism of Aconitine Kusnezoffii Radix Processed with Chebulae Fructus against H9c2 Cardiomyocyte Toxicity Based on TRPV1 Channel. Chin. J. Exp. Traditional Med. Formulae, 2022, 173–181. doi:10.13422/j.cnki.syfjx.20211748
Hu, T., Tang, X., Wen, X., Xie, X., Peng, C., and Rao, C. (2018). Study the Effects and Mechanism of Autophagy in H9C2 Cardiomyocytes Apoptosis Induced by Aconitine. J. Chin. Med. Mater. 41, 1191–1196. doi:10.13863/j.issn1001-4454.2018.05.039
Kang, J. X., and Leaf, A. (1996). Evidence that Free Polyunsaturated Fatty Acids Modify Na+ Channels by Directly Binding to the Channel Proteins. Proc. Natl. Acad. Sci. U. S. A. 93, 3542–3546. doi:10.1073/pnas.93.8.3542
Li, C. Y., Zhou, Z., Xu, T., Wang, N. Y., Tang, C., Tan, X. Y., et al. (2022). Aconitum Pendulum and Aconitum Flavum: A Narrative Review on Traditional Uses, Phytochemistry, Bioactivities and Processing Methods. J. Ethnopharmacol. 292, 115216. doi:10.1016/j.jep.2022.115216
Li, H., Liu, L., Zhu, S., and Liu, Q. (2016). Case Reports of Aconite Poisoning in Mainland China from 2004 to 2015: A Retrospective Analysis. J. Forensic Leg. Med. 42, 68–73. doi:10.1016/j.jflm.2016.05.016
Li, M., Xie, X., Chen, H., Xiong, Q., Tong, R., Peng, C., et al. (2020). Aconitine Induces Cardiotoxicity through Regulation of Calcium Signaling Pathway in Zebrafish Embryos and in H9c2 Cells. J. Appl. Toxicol. 40, 780–793. doi:10.1002/jat.3943
Li, M., Ren, S., Xu, H., Wang, Y., Ma, Z., and Gao, Y. (2018). Effects of Aconitine on ATPase and Related Ions in Cardiac Myocytes. Pharmacol. Clin. Chin. Materia Medica 34, 25–28. doi:10.13412/j.cnki.zyyl.2018.02.007
Liang, Q., Liu, M., and Hu, B. (1991). The Histo-And Cyto-Chemdcal Demonstration of NADH Dehydrogenasein Myocardium of Aconitine Poisoning Rat. Chin. J. Forensic Med., 15, 84–86+133. doi:10.13618/j.issn.1001-5728.1991.02.009
Lin, C. C., Chan, T. Y., and Deng, J. F. (2004). Clinical Features and Management of Herb-Induced Aconitine Poisoning. Ann. Emerg. Med. 43, 574–579. doi:10.1016/j.annemergmed.2003.10.046
Liu, F., Han, X., Li, N., Liu, K., and Kang, W. (2019). Aconitum Alkaloids Induce Cardiotoxicity and Apoptosis in Embryonic Zebrafish by Influencing the Expression of Cardiovascular Relative Genes. Toxicol. Lett. 305, 10–18. doi:10.1016/j.toxlet.2019.01.002
Liu, Y. (2007). Myocardial Toxicity of Aconite Alkaloids. Beijing, China: Shenyang Pharmaceutical University. Master Thesis. CNKI online database. Available at: https://chn.oversea.cnki.net/index/.
Liu, Y. (2009). Toxic Effects of Aconitine in Rats' Cardiomyocytes: A Study on Molecular Toxicology Mechanism. Beijing, China: Huazhong University of Science and Technology. Master Thesis. CNKI online database. Available at: https://chn.oversea.cnki.net/index/.
Meng, Q. (2006). Toxic Effect of Aconitine on Myocardial Cells. Beijing, China: Chengdu University of Traditional Chinese Medicine. Master Thesis. CNKI online database. Available at: https://chn.oversea.cnki.net/index/.
Peng, F., Zhang, N., Wang, C., Wang, X., Huang, W., Peng, C., et al. (2020). Aconitine Induces Cardiomyocyte Damage by Mitigating BNIP3-dependent Mitophagy and the TNFα-NLRP3 Signalling axis. Cell. Prolif. 53, e12701. doi:10.1111/cpr.12701
Sena, E., Van Der Worp, H. B., Howells, D., and Macleod, M. (2007). How Can We Improve the Pre-clinical Development of Drugs for Stroke? Trends Neurosci. 30, 433–439. doi:10.1016/j.tins.2007.06.009
Singhuber, J., Zhu, M., Prinz, S., and Kopp, B. (2009). Aconitum in Traditional Chinese Medicine: a Valuable Drug or an Unpredictable Risk? J. Ethnopharmacol. 126, 18–30. doi:10.1016/j.jep.2009.07.031
Sun, G. B., Sun, H., Meng, X. B., Hu, J., Zhang, Q., Liu, B., et al. (2014). Aconitine-induced Ca2+ Overload Causes Arrhythmia and Triggers Apoptosis through P38 MAPK Signaling Pathway in Rats. Toxicol. Appl. Pharmacol. 279, 8–22. doi:10.1016/j.taap.2014.05.005
Wang, M., Shi, Y., Yao, L., Li, Q., Wang, Y., and Fu, D. (2020a). Potential Molecular Mechanisms and Drugs for Aconitine-Induced Cardiotoxicity in Zebrafish through RNA Sequencing and Bioinformatics Analysis. Med. Sci. Monit. 26, e924092. doi:10.12659/msm.924092
Wang, S., Chen, A., and Li, F. (2020b). Research Advances of Cardiotoxicity Mechanism of Aconitine-Induce Arrhythmia Advances. Northwest Pharm. J. 35, 972–975. doi:10.3969/j.issn.1004-2407.2020.06.039
Wang, S., Hou, Y., Xing, N., Meng, X., Zhang, Y., and Wang, X. (2022a). Identification of a Novel Prognostic Signature Related to PANoptosis and its Regulatory Mechanism as Well as Targeted Treatment of Active Ingredients and Traditional Chinese Medicine in Lung Adenocarcinoma. Pharmacol. Res. - Mod. Chin. Med. 2, 100069. doi:10.1016/j.prmcm.2022.100069
Wang, W., Jiang, J., Huang, Y., Peng, F., Hu, T., Wu, J., et al. (2022b). Aconitine Induces Autophagy via Activating Oxidative DNA Damage-Mediated AMPK/ULK1 Signaling Pathway in H9c2 Cells. J. Ethnopharmacol. 282, 114631. doi:10.1016/j.jep.2021.114631
Wang, X., Hou, Y., Li, Q., Li, X., Wang, W., Ai, X., et al. (2019a). Rhodiola Crenulata Attenuates Apoptosis and Mitochondrial Energy Metabolism Disorder in Rats with Hypobaric Hypoxia-Induced Brain Injury by Regulating the HIF-1α/microRNA 210/ISCU1/2(COX10) Signaling Pathway. J. Ethnopharmacol. 241, 111801. doi:10.1016/j.jep.2019.03.028
Wang, X. C., Jia, Q. Z., Yu, Y. L., Wang, H. D., Guo, H. C., Ma, X. D., et al. (2021). Inhibition of the INa/K and the Activation of Peak INa Contribute to the Arrhythmogenic Effects of Aconitine and Mesaconitine in guinea Pigs. Acta Pharmacol. Sin. 42, 218–229. doi:10.1038/s41401-020-0467-6
Wang, X. (2013). Comparsion of the Arrhythmogenic Effects between ACO and MACO on Ventricular Myocytes of guinea-pig and Their Underlying Cellular Mechanisms. Beijing, China: Hebei Medical University. Master Thesis. CNKI online database. Available at: https://chn.oversea.cnki.net/index/.
Wang, X., Liu, Z., Fan, F., Hou, Y., Yang, H., Meng, X., et al. (2019b). Microfluidic Chip and its Application in Autophagy Detection. TrAC Trends Anal. Chem. 117, 300–315. doi:10.1016/j.trac.2019.05.043
Wang, Y. (2007). Aconitine Toxic Chinese Medicine Toxic Components on Myocardial Cell Membrane Receptor. Beijing, China: Chengdu University of Traditional Chinese Medicine. Master thesis. CNKI online database. Available at: https://chn.oversea.cnki.net/index/.
Wang, Y., Li, H., Zhang, J., Wang, J., and Wang, L. (2007). Toxicity Effects of Aconitine on Neonatal Rat Cardiomyocytes. West China J. Pharm. Sci. 5, 4–6. doi:10.13375/j.cnki.wcjps.2007.01.002
Xia, Q., Gao, S., Rapael Gnanamuthu, S. R., Zhuang, K., Song, Z., Zhang, Y., et al. (2021). Involvement of Nrf2-HO-1/jnk-Erk Signaling Pathways in Aconitine-Induced Developmental Toxicity, Oxidative Stress, and ROS-Mitochondrial Apoptosis in Zebrafish Embryos. Front. Pharmacol. 12, 642480. doi:10.3389/fphar.2021.642480
Xiong, L., Zhang, J. Z., He, R., and Hamilton, S. L. (2006). A Ca2+-Binding Domain in RyR1 that Interacts with the Calmodulin Binding Site and Modulates Channel Activity. Biophys. J. 90, 173–182. doi:10.1529/biophysj.105.066092
Xu, G. (2008). Study on DNA Damage and Mechanism in Primary Cardiomyocytes Caused by Aconitine. Beijing, China: The Second Affiliated Hospital of Henan University of traditional Chinese Medicine. Master Thesis. CNKI online database. Available at: https://chn.oversea.cnki.net/index/.
Yamamoto, T., El-Hayek, R., and Ikemoto, N. (2000). Postulated Role of Interdomain Interaction within the Ryanodine Receptor in Ca(2+) Channel Regulation. J. Biol. Chem. 275, 11618–11625. doi:10.1074/jbc.275.16.11618
Yang, C., Zeng, X., Cheng, Z., Zhu, J., and Fu, Y. (2021). Aconitine Induces TRPV2-Mediated Ca2+ Influx through the P38 MAPK Signal and Promotes Cardiomyocyte Apoptosis. Evid. Based Complement. Altern. Med. 2021, 9567056. doi:10.1155/2021/9567056
Ye, Q., Liu, H., Fang, C., Liu, Y., Liu, X., Liu, J., et al. (2021). Cardiotoxicity Evaluation and Comparison of Diterpene Alkaloids on Zebrafish. Drug Chem. Toxicol. 44, 294–301. doi:10.1080/01480545.2019.1586916
Yu, C. (2015). Spontaneous Calcium Release Induced by Dieste-Aconite Alkaloid on Cardiac Myocytes. Beijing, China: China Academy of Chinese Medical Sciences. CNKI online database. Available at: https://chn.oversea.cnki.net/index/
Zhang, B., Chen, L., Ran, F., Du, K., Yu, F., Chen, W., et al. (2020). Effects of Aconitine on H9c2 Cell Differentiation. Anhui Med. Pharm. Journa 24, 1287–1290+1485. doi:10.3969/j.issn.1009⁃6469.2020.07.004
Zhang, L., Tang, X., Yao, M., Liu, Y., and Zhao, F. (2018). Investigation of the Toxicity of Three Alkaloids in Junggar Aconiyumon H9c2 Cells. J. Xinjiang Univ. 41, 1391–1395. doi:10.3969/j.issn.1009-5551.2018.11.017
Zhang, S. (2007). Effects of Aconitine on Connexin43 Phosphorylation Status and [Ca2+] Oscillation Patterns in Cultured Neonatal Rat Ventricular Myocytes. Beijing, China: Huazhong University of Science and Technology. Master Thesis. CNKI online database. Available at: https://chn.oversea.cnki.net/index/.
Zhang, S., Wu, J., Jia, Z., and Sun, J. (2012). Dose-toxicity Relationship Study for Cardiotonic Effect of Aconitine. Chin. J. Exp. Traditional Med. Formulae 18, 222–225. doi:10.13422/j.cnki.syfjx.2012.19.064
Zhang, X., Cui, Y., Miao, X., Liu, D., Ma, Z., and Li, G. (2017). Protective Effect of Mongolian Medicine Terminalia Chebula on Cardiotoxicity Induced by Aconium Kusnezoffii in Rats. J. Chin. Med. Mater., 34, 2693–2696. doi:10.13863/j.issn1001-4454.2017.11.044
Zhou, G., Tang, L., Zhou, X., Wang, T., Kou, Z., and Wang, Z. (2015). A Review on Phytochemistry and Pharmacological Activities of the Processed Lateral Root of Aconitum Carmichaelii Debeaux. J. Ethnopharmacol. 160, 173–193. doi:10.1016/j.jep.2014.11.043
Zhou, S. S., Yang, J., Li, Y. Q., Zhao, L. Y., Xu, M., and Ding, Y. F. (2005). Effect of Cl- Channel Blockers on Aconitine-Induced Arrhythmias in Rat Heart. Exp. Physiol. 90, 865–872. doi:10.1113/expphysiol.2005.031484
Zhou, W., Liu, H., Qiu, L. Z., Yue, L. X., Zhang, G. J., Deng, H. F., et al. (2021). Cardiac Efficacy and Toxicity of Aconitine: A New Frontier for the Ancient Poison. Med. Res. Rev. 41, 1798–1811. doi:10.1002/med.21777
Zhou, W., Qiu, L. Z., Liu, H., Deng, H. F., Yue, L. X., and Gao, Y. (2020). Notch1-mediated Histone Demethylation of HCN4 Contributes to Aconitine-Induced Ventricular Myocardial Dysrhythmia. Toxicol. Lett. 327, 19–31. doi:10.1016/j.toxlet.2020.03.017
Keywords: aconitine, cardiotoxicity, preclinical evidence, ion channels, mitochondrial damage, NLRP3
Citation: Jiang H, Zhang Y, Zhang Y, Wang X and Meng X (2022) An Updated Meta-Analysis Based on the Preclinical Evidence of Mechanism of Aconitine-Induced Cardiotoxicity. Front. Pharmacol. 13:900842. doi: 10.3389/fphar.2022.900842
Received: 22 March 2022; Accepted: 02 May 2022;
Published: 08 June 2022.
Edited by:
Rolf Teschke, Hospital Hanau, GermanyReviewed by:
Bunleu Sungthong, Mahasarakham University, ThailandVincenzo Quagliariello, G. Pascale National Cancer Institute Foundation (IRCCS), Italy
Copyright © 2022 Jiang, Zhang, Zhang, Wang and Meng. This is an open-access article distributed under the terms of the Creative Commons Attribution License (CC BY). The use, distribution or reproduction in other forums is permitted, provided the original author(s) and the copyright owner(s) are credited and that the original publication in this journal is cited, in accordance with accepted academic practice. No use, distribution or reproduction is permitted which does not comply with these terms.
*Correspondence: Xianli Meng, xlm999@cdutcm.edu.cn; Xiaobo Wang, VitaDrwang@cdutcm.edu.cn; Yi Zhang, zhangyi@cdutcm.edu.cn