- 1State Key Laboratory of Southwestern Chinese Medicine Resources, School of Ethnic Medicine, Chengdu University of Traditional Chinese Medicine, Chengdu, China
- 2State Key Laboratory of Southwestern Chinese Medicine Resources, Research Center for Academic Inheritance and Innovation of Ethnomedicine, Chengdu University of Traditional Chinese Medicine, Chengdu, China
- 3State Key Laboratory of Southwestern Chinese Medicine Resources, School of Pharmacy, Chengdu University of Traditional Chinese Medicine, Chengdu, China
Background: Herbs originating from the Aconitum L. (Ranunculaceae), such as Aconitum carmichaelii Debeaux. (Wutou), Aconitum pendulum Busch. (Tiebangchui), and Aconitum kusnezoffii Reichb. (Caowu), etc. are highly valued for their medicinal properties. The roots and tubers of these herbs are commonly used to treat an array of ailments, including joint pain and tumors. The alkaloids present in them are the primary active components, with aconitine being the most notable. Aconitine has gained attention for its exceptional anti-inflammatory and analgesic properties, as well as its potential as an anti-tumor and cardiotonic agent. However, the exact process through which aconitine hinders the growth of cancerous cells and triggers their programmed cell death remains unclear. Therefore, we have undertaken a comprehensive systematic review and meta-analysis of the current research on the potential antitumor properties of aconitine.
Methods: We conducted a thorough search of relevant preclinical studies in databases including PubMed, Web of Science, VIP, WanFang Data, CNKI, Embase, Cochrane Library, and National Center for Biotechnology Information (NCBI). The search was conducted up until 15 September 2022, and the data were statistically analyzed using RevMan 5.4 software. The number of tumor cell value-added, tumor cell apoptosis rate, thymus index (TI), and Bcl-2 gene expression level were the main indicators to be analyzed.
Results: After applying the final inclusion criteria, a total of thirty-seven studies, comprising both in vivo and in vitro research were analyzed. The results showed that treatment with aconitine led to a significant reduction in tumor cell proliferation, a noteworthy increase in the rate of apoptosis among tumor cells, a decrease in the thymus index, and a reduction in the expression level of Bcl-2. These results suggested that aconitine could inhibit the proliferation, invasion, and migration abilities of tumor cells by regulating Bcl-2 etc., thereby enhancing the anti-tumor effects.
Conclusion: In summary, our present study demonstrated that aconitine effectively reduced tumor size and volume, indicating a strong anti-tumor effect. Additionally, aconitine could increase the expression levels of caspase-3, Bax and other targets. Mechanistically, it may regulate the expression levels of Bax and Bcl-2 through the NF-κB signaling pathway, ultimately inhibiting tumor cell proliferation through autophagy.
1 Introduction
A malignant tumor refers to a vast array of diseases that are characterized by the loss of normal cellular regulation, uncontrolled growth, abnormal differentiation, local tissue infiltration, and distant transplantation (Reardon, 2010). Malignant tumors can develop in any tissue of any organ and can occur at any age (Klein and Vande, 2008). The International Agency for Research on Cancer (IARC) of the World Health Organization has released the latest global cancer burden data, revealing that there will be 19.29 million new cancer cases worldwide in 2020. Shockingly, 4.57 million of these cases will be in China, accounting for 23.7% of the new global cancer cases. To make matters worse, both the number of cancer deaths and the number of new cancers globally rank first in the world (Zhang, 2021). In the field of oncology, there are several options available to treat malignant tumors. These include surgery, radiotherapy (Baskar et al., 2012), chemotherapy, immunotherapy, targeted therapy (Guan et al., 2018), endocrine therapy (Reinbolt et al., 2015), stem cell transplantation (Hawsawi et al., 2018), and DNA precision therapy (Raimundo et al., 2021). However, two of the most commonly used treatments, chemotherapy and radiotherapy, often come with a range of side effects (Tommelein et al., 2018), such as damage to local radiation areas, hair loss, nausea and vomiting, fever, and impaired hematopoietic function. Additionally, oral drugs used in treatment can also lead to reduced patient wellbeing due to associated side effects (Lin et al., 2017; Ding and Lu, 2019). With targeted therapy, the required drugs are taken for a long time and the specific drugs are expensive. Therefore, it is crucial to find alternative drugs for the treatment of malignant tumors. The current treatment modalities for malignancies are shown in Figure 1A. Chinese herbal medicine has become a Frontier area of oncology drug research because of its lesser side effects and unique pharmacological activities. More and more scholars have started to search for natural products with antitumor efficacy from herbal medicines.
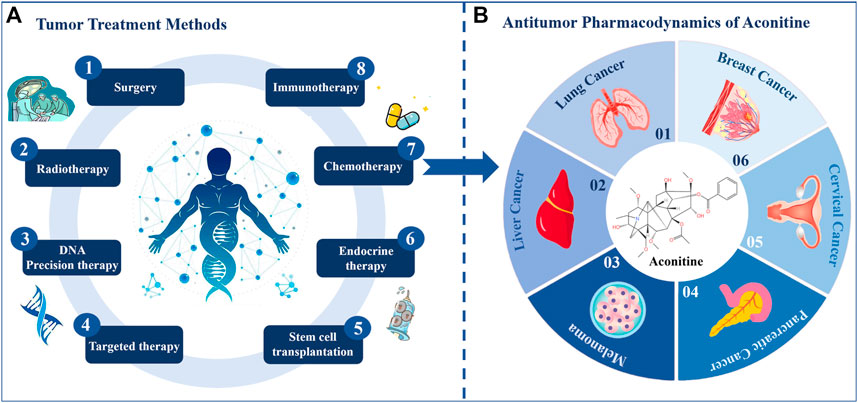
FIGURE 1. The main method of tumor treatment. (A) Tumor treatment methods. (B) Antitumor pharmacodynamics of aconitine.
Medicinal materials derived from Aconitum L., including Aconitum carmichaelii Debeaux. (Wutou), Aconitum brachypodum Diels. (Xueshangyizhihao), Aconitum coreanum (H. Lév.) Raipaics. (Huanghuawutou), Aconitum kusnezoffii Reichb. (Caowu), and Aconitum pendulum Busch. (Tiebangchui), etc. are well-known Chinese herbal medicines. It is reported to be widely used in many traditional medical systems, including Chinese, Tibetan, Mongolian, and Indian medicine. Modern pharmacological research has confirmed their analgesic and anti-tumor effects, which has sparked interest among scholars in the field of oncology (Ma et al., 2015; Li et al., 2022) (Figure 2). Aconitine (C34H47NO11) is a C19-diterpenoid alkaloid that possesses good anti-tumor effects and is the main medicinal ingredient in Aconitum medicinal materials (Yang et al., 2016) (Figure 1B). Clinically, aconitine is mostly used to prevent and treat cancer, including pancreatic cancer (Ji et al., 2016), ovarian cancer (Li et al., 2018), breast cancer (Guo et al., 2011), lung cancer (Zhang et al., 2020), liver cancer (Qi et al., 2018a; Yao et al., 2021) and melanoma (Du J et al., 2013), etc. Its action mechanism is primarily to induce apoptosis, inhibit cancer cell proliferation and migration (Garmanchouk et al., 2005; Wang et al., 2020; Wang et al., 2022), etc. In addition, it is also used for local anesthesia (Chan et al., 2021), analgesia (Wang et al., 2008), anti-inflammation (Zheng et al., 2017), and sweating (Wu et al., 2021; Zhang et al., 2021). Aconitine has shown excellent efficacy in anti-inflammation, for instance, in the treatment of rheumatoid arthritis, by regulating IL-6 and TNF-α cytokine levels and inhibiting the activation of NF-κB signaling pathway (Chen et al., 2021). Currently, several animal and cellular experiments have confirmed the inhibitory effect of aconitine alkaloids on malignant tumors (Yao et al., 2021; Li et al., 2022). As shown above, aconitine shows powerful antitumor potential in a variety of tumors and has good prospects for development and application.
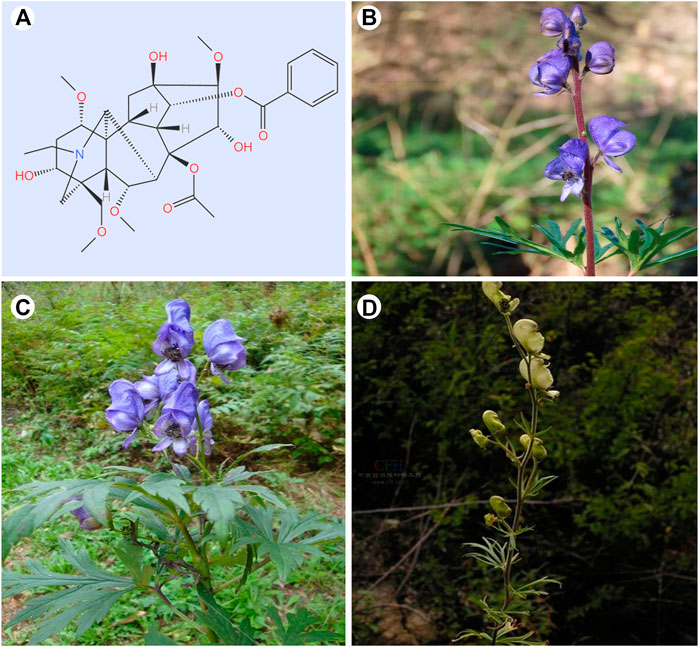
FIGURE 2. Structural formula of aconitine and botanical diagram of some major Aconitum source species. (A) The structural formula of Aconitine. (B) Aconitum carmichaelii Debeaux. (Wutou). (C) Aconitum brachypodum Diels. (Xueshangyizhihao). (D) Aconitum coreanum (H. Lév.) Raipaics. (Huanghuawutou). The representative photos of the three Aconitum species are from the website: https://www.gbif.org/; http://powo.science.kew.org.
Although aconitine has anti-inflammatory and analgesic properties, improper use can result in severe cardiac arrhythmias, shock, and coma (Gao et al., 2020). This is because aconitine affects voltage-sensitive sodium channels in excitable tissues such as cardiac muscle, nerve, and muscle, leading to cardiotoxicity and neurotoxicity (Yang et al., 2021; Gao et al., 2022; Jiang et al., 2022). Furthermore, studies in recent years have also shown that aconitine can cause embryotoxicity (Li et al., 2020), nephrotoxicity (Jin et al., 2020), hepatotoxicity (Ji et al., 2019), and reproductive toxicity (Wang et al., 2019). Numerous preclinical studies have demonstrated that aconitine has a notable inhibitory effect on malignant tumors. However, these results originate from various laboratories and users, which may result in some inaccuracies. As a result, it is essential to integrate and analyze the study outcomes using appropriate methods.
Systematic review is a highly effective method of producing dependable information that can serve as the most authoritative form of medical evidence. According to the Oxford Center for Evidence-Based Medicine grades of evidence, only data from systematic reviews can be recognized as 1A evidence (Glasziou et al., 2004). As a generally accepted and effective method, the search for aconitine’s extensive preclinical evidence of inhibiting malignant tumors and promoting apoptosis of malignant tumor cells in vitro and in vivo can help expand the clinical application of aconitine and the subsequent development of related anticancer drugs. In this study, a systematic evaluation and meta-analysis of aconitine’s antitumor properties were conducted for the first time. This involved searching both domestic and international literature databases to identify relevant preclinical literature and to determine the anti-tumor mechanism of aconitine. The aim was to provide a systematic evaluation that would serve as an evidence-based foundation for subsequent anti-tumor research on aconitine.
2 Materials and methods
2.1 Protocol
This meta-analysis strictly adhered to the protocol registered in PROSPERO (CRD42022370809) and followed the PRISMA guidelines.
2.2 Retrieval strategy
In this subject paper, we utilized various computerized search databases such as WanFang Data, CNKI, VIP, PubMed, Embase, Web of Science, and Cochrane Library. The search period was limited to the date of creation up to 15 September 2022. Additionally, to ensure that we did not miss any relevant articles, we conducted a manual search of the retrieved articles.
2.2.1 Chinese database retrieval strategy
The Chinese literature search was conducted through the following search strategy: ① “aconitine” or “aconitine alkaloid”; ② “anti-cancer” or “anti-tumor” or “anti-proliferation” or “anti-proliferative” or “anti-proliferative activity” or “inhibition of cell proliferation”; ③ “cancer cell” or “tumor cell”; ④ including ①②③ and other subject words or free words.
The search strategy for Chinese literature involved the following steps: ①, searching for keywords “aconitine” or “aconitine alkaloid”; ②, looking for terms such as “anti-cancer” or “anti-tumor” or “anti-proliferation” or “anti-proliferative” or “anti-proliferative activity” or “inhibition of cell proliferation”; ③, searching for phrases like “cancer cell” or “tumor cell”; ④, including ①②③ along with other relevant subject words or free words.
2.2.2 English database retrieval strategy
To search for English literature on the topic, the following strategy was employed: ①, Keywords such as “Aconitine” or “Aconitum alkaloid” or “Aconitine alkaloids” were used; ②, Terms such as “Anticancer” or “Antitumor” or “Antiproliferative” “Anti-proliferation” or “Antiproliferative activity” were included; ③, Phrases like “Cancer cell” or “Tumor cell” were also added; ④, All the above terms were combined using “OR” and “AND” to form a comprehensive search group. In addition, other relevant subject words and free words were included.
These were the techniques we used to conduct our literature searches: [“aconitine” (MeSH Terms) OR “aconitine” (All Fields)] OR [“aconitum” (MeSH Terms) OR “aconitum” (All Fields)] AND [“alkaloids” (MeSH Terms) OR “alkaloids” (All Fields) OR “alkaloid” (All Fields)] OR [“aconitine” (MeSH Terms) OR “aconitine” (All Fields)] AND [“alkaloids” (MeSH Terms) OR “alkaloids” (All Fields)] AND [Anticancer (All Fields) OR Antitumor (All Fields) OR Antiproliferative (All Fields) OR Anti-proliferation (All Fields)] OR [Antiproliferative (All Fields)] AND [“motor activity” (MeSH Terms)] OR [“motor” (All Fields) AND “activity” (All Fields)] OR [“motor activity” (All Fields) OR “activity” (All Fields)] AND [“cancer” (All Fields) AND “cell” (All Fields)] OR [“cancer cell” (All Fields)] OR [“tumour” (All Fields) OR “neoplasms” (MeSH Terms) OR “neoplasms” (All Fields) OR “tumor” (All Fields)] AND [“cells” (MeSH Terms) OR “cells” (All Fields) OR “cell” (All Fields)].
2.3 Literature inclusion and exclusion criteria
2.3.1 Inclusion criteria
1) Study: Aconitine inhibits malignant tumor cell proliferation and promotes tumor cell apoptosis related article. 2) The experimental group received aconitine monotherapy without regard to treatment method, dose and frequency. 3) The control group was given only saline, pure water or no adjuvant intervention. 4) Outcome indicators: the main outcome indicators included tumor weight, tumor cell inhibition rate (IR), tumor cell apoptosis rate, thymus index (TI), and degree of apoptotic invasion, which encompassed at least one of the above indicators.
2.3.2 Exclusion criteria
1) The target disease was not malignancy, cancer, etc.; 2) There was no control group; 3) The trial group received a combination of aconitine; 4) Duplicate published literature; 5) Studies were clinical studies, case reports, clinical trials, reviews, conference papers, abstracts, reviews, and patent results; 6) Unpublished dissertations; and 7) Literature for which data could not be extracted.
2.3.3 Literature screening and data extraction
Two investigators conducted literature screening and data extraction independently, utilizing Endnote and adhering to the aforementioned inclusion and exclusion criteria. The extracted data will be organized using Excel software and cross-checked for accuracy. In the event of any discrepancies, a joint decision will be made after consulting with a third researcher. Further details regarding the data extraction process can be found below: 1) The first author and year of publication of the literature; 2) Individual data of the experimental study, such as animal species, sex, body weight, and individual comparison samples; 3) The type of anesthesia; 4) The intervention characteristics of the treatment and control groups, including drug dosage form, dose, treatment method, and frequency of administration; and 5) The mean, standard deviation, and between-group differences of measurements and corresponding data.
2.3.4 Quality evaluation of included documents
Two authors conducted independent assessments of the methodological quality of the studies included in this paper. The assessments were based on the CAMARADES list, which provides a collaborative approach to meta-analysis and review of animal data from experimental studies. The list comprises 10 items (Sena et al., 2007): ①Publication of the paper after peer review; ② Description of temperature control; ③ Randomization to treatment and control groups; ④ Blinding of the model; ⑤ Blinded assessment of the results; ⑥ No intrinsic neuroprotective effect of the use of anesthetics; ⑦ Appropriate animal models; ⑧ Sample size calculation; ⑨ Compliance with animal protection regulations, and ⑩ Declaration of any potential conflicts of interest. Each project was rated for overall quality using a single-point system. In the event of any discrepancies in the quality assessment, a third investigator was consulted to resolve them.
2.3.5 Statistical methods
Meta-analysis was performed by using Review Manager 5.4 software. Odds ratios (OR) were used to express count data as statistical effect sizes, while mean difference (MD) was used to express continuous variables. To ensure accuracy, all effect sizes were accompanied by a 95% confidence interval (CI). Heterogeneity was evaluated using Higgins I2, and when the results of the heterogeneity test were not statistically significant (p > 0.05, I2 ≤ 50%), a fixed-effects model was employed for meta-analysis, However, if I2 > 50%, a heterogeneity test was required to identify the cause of heterogeneity. If heterogeneity remained above 50% after sensitivity analysis or subgroup analysis, only a description of the results was required. The results were considered statistically significant with a p-value of p < 0.05. Meta-analysis test level was a = 0.05, and publication bias was expressed using funnel plots.
3 Results
3.1 Study inclusion
Based on the search method described above, a total of 2581 documents were initially searched. After removing 407 duplicate documents, 2174 articles remained. Of these, 1606 articles were excluded as they were reviews, experiences, conferences, patents, or results, leaving 568 articles after the initial screening. After reviewing the titles and abstracts of these articles, 450 papers were found to have inconsistent contents and were excluded, resulting in a final selection of 118 papers. After reading the full text, we excluded several papers. Specifically, we excluded 21 papers that were reviewed, 36 papers that involved combined drug interventions in both the treatment and control groups, 21 papers from which we were unable to extract experimental data, 2 clinical papers, 8 papers that were tested with drugs other than aconitine alkaloids, and 30 papers that studied diseases other than antitumor. Ultimately, we included 37 literatures for analysis. The screening process is shown in Figure 3. Included literature is shown in Table 1.
3.2 Basic characteristics of included literature
The subject paper included thirty-seven preclinical studies, which could be classified into five animal experiments, twenty-four cellular experiments, and eight experiments that involved both animal and cellular testing. The animal experiments involved a combination of male and female animals in four studies, while eight studies used only male animals and one study used only female animals. Additionally, thirteen animal studies provided information on the body weight of the animals. Eight studies did not report the method of animal execution, while four studies utilized decerebrate execution and one study used 0.1 mL of 1% pentobarbital solution for anesthetic execution. The dosing time ranged from a minimum of 5 days to a maximum of 26 weeks. In terms of cellular experiments, fifteen studies mentioned the cell inhibition rate or cell proliferation inhibition rate. Sixteen studies reported on apoptosis rate, while six studies focused on detecting rate OD or absorbance A value. Additionally, five studies provided insight into different cell cycle numbers, while six studies explored cell invasion ability, cell invasion inhibition rate, or cell invasion number. Furthermore, twelve studies delved into the expression levels of proteins such as p21-Ras, p38MAPK, p53, Bcl-2, Bax, and others. The detailed characteristics of the included studies are shown in Table 2 and Table 3.
3.3 Included in literature quality evaluation
The CAMARADES evaluation form was utilized to assess the quality of the literature included in the 37 preclinical trials, as presented in Table 4. The studies were evaluated on a scale of 4-7, with an average score of 5.32. Among these studies, 4 (Zhao et al., 2014; Zhang et al., 2017; Wu et al., 2018; Cheng et al., 2020); 10 (Zeng et al., 2007; Rao and Peng, 2010; Liu, 2013; Ji et al., 2016; Ma, 2017; Qi et al., 2018b; Cheng, 2019; Wang, 2020; Wang et al., 2020; Luan et al., 2022) studies received 6 points; 18 (Xu et al., 2008; Zhu, 2008; Jia and Zhang, 2011; Zhang and Wu, 2011; Ding et al., 2013; Ding, 2014; Guan et al., 2015; Fan et al., 2016; Guan et al., 2017; Ma and Yu, 2018; Xiong et al., 2018; Zhang et al., 2018; Shao et al., 2019; Zhou et al., 2019; Ru et al., 2020; Zhang et al., 2020; Zou et al., 2021; Cai, 2022) studies received 5 points; 5 (Tang and Sun, 1986; Yang et al., 2005; Ren and Zeng, 2008; Wuliji et al., 2008; Hao, 2014) studies received 4 scores. It is worth noting that all of these studies went through the peer review process before publication. While all studies were randomized, 11 of them (Tang and Sun, 1986; Yang et al., 2005; Zeng et al., 2007; Ren and Zeng, 2008; Wuliji et al., 2008; Rao and Peng, 2010; Hao, 2014; Zhang et al., 2017; Wu et al., 2018; Cheng, 2019; Wang, 2020) failed to provide an accurate description of their grouping method, and 3 (Tang and Sun, 1986; Yang et al., 2005; Ren and Zeng, 2008) studies did not mention temperature control. Additionally, none of the studies mentioned implementing model-blinded or outcome-blinded methods. 9 (Zeng et al., 2007; Ren and Zeng, 2008; Rao and Peng, 2010; Zhao et al., 2014; Zhang et al., 2017; Wu et al., 2018; Cheng, 2019; Cheng et al., 2020; Wang, 2020) studies were found to mention lethal mode or anesthetic use. All of these studies utilized appropriate animal or cellular models and calculated the necessary data. 12 (Tang and Sun, 1986; Yang et al., 2005; Zeng et al., 2007; Rao and Peng, 2010; Liu, 2013; Zhao et al., 2014; Ma, 2017; Zhang et al., 2017; Wu et al., 2018; Cheng, 2019; Cheng et al., 2020; Wang, 2020) studies mentioned animal welfare regulations, and only 6 (Ji et al., 2016; Zhang et al., 2017; Qi et al., 2018b; Wu et al., 2018; Wang et al., 2020; Luan et al., 2022) studies included a statement regarding potential conflicts of interest.
3.4 Meta analysis results
3.4.1 Tumor weight
Seven studies were analyzed to compare the change in tumor weight between the control and experimental groups. Heterogeneity was observed between the (Tau2 = 0.28, Chi2 = 73.07, df = 6, I2 = 92%, p < 0.00001), so a random-effects model was utilized. The findings indicated a significant difference in tumor weight between the treatment and control groups (Z = 5.81, p < 0.00001) with a 95% confidence interval of [MD = −1.28, 95% CI (−1.72, −0.85)]. The results were shown in Figure 4.
3.4.2 Proliferation number of tumor cells
Cell proliferation is a crucial aspect of an organism’s life, occurring through cell division. Protozoans generate new individuals through cell division, while multicellular organisms produce new cells to replace aging or dying cells within the body (Ji, 2021). Cancer cells, on the other hand, possess three significant traits: infinite proliferation (Jarrett et al., 2018), the ability to transform and metastasize, and the capacity to destroy normal cellular tissues. Therefore, the value-added number of tumor cells is one of the important indicators for the evaluation of antitumor efficacy (Kotel'Nikov, 1989).
Two studies were analyzed to compare changes in cell proliferation numbers between control and experimental groups. Heterogeneity was observed between trials (Tau2 = 0.06, Chi2 = 166.34, df = 1, I2 = 99%, p < 0.00001), requiring the use of a random effects model. The results indicated a significant difference between the control and experimental groups (Z = 3.84, p = 0.0001) with a 95% confidence interval of [MD = −0.64, 95% CI (−0.97, −0.31)]. This indicated that aconitine could reduce the number of tumor cell proliferation in mice. The results were shown in Figure 5.
3.4.3 Thymus index
The thymic index, also referred to as the thymic secretion index, is primarily based on the level of lymphocyte multiplication and can serve as a means to gauge immune intensity (Kubatka et al., 2019).
A total of two studies were included to compare the change in thymic index between the control and experimental groups. Due to heterogeneity between trials (Chi2 = 10.56, df = 1, I2 = 91%, p = 0.001), a random-effects model was utilized. The results showed a statistically significant difference in thymic index between the treatment and control groups (Z = 39.30, p < 0.00001), with a 95% confidence interval of [MD = −0.61, 95% CI (−0.64, −0.58)]. The results of this study showed that aconitine could reduce the thymic index in mice. The results were shown in Figure 6.
3.4.4 Number of metastatic lesions
Metastatic lesions refer to tumor cells that spread from the primary site through lymphatic vessels, blood vessels, or other pathways to other areas of the body where they continue to grow, forming the same type of tumor as the primary site. This process is known as metastasis, and the resulting tumors are called metastases or metastatic cancer (Suhail et al., 2019). The evaluation of the number of metastatic lesions is frequently used in antitumor studies because of the susceptibility of tumor cells to metastasize.
A total of two studies were included to compare the changes in the number of metastatic lesions between the control and experimental groups, and there was heterogeneity between the trials (Tau2 = 79.22, Chi2 = 56.40, df = 1, I2 = 98%, p < 0.00001), so a random-effects model was used. T The results indicated that there was no significant difference in the number of metastatic lesions between the treatment and control groups (Z = 1.33, p = 0.18). Therefore, it cannot be concluded that aconitine has the ability to reduce the number of metastatic lesions in mice. The results were shown in Figure 7.
3.4.5 Tumor cell apoptosis rate
Apoptosis is a genetically controlled process of autonomous and orderly cell death that helps maintain the stability of the internal environment (Elmore, 2007). In contrast, cancer cells can evade apoptosis and grow uncontrollably. As a result, the effectiveness of drugs in treating tumors is frequently evaluated by measuring the apoptosis rate of cancer cells.
A total of seven studies were included to compare apoptosis rates between control and experimental groups, and there was heterogeneity between trials (Tau2 = 382.07, Chi2 = 40,694.89, df = 6, I2 = 100%, p < 0.00001), so a random-effects model was used. The results indicated a significant difference in apoptosis rate between the treatment and control groups (Z = 4.14, p < 0.0001) with a 95% confidence interval of [MD = 30.62,95% CI (16.13, 45.11)]. The results of this study showed that aconitine could increase the apoptosis rate of tumor cells. The results were shown in Figure 8.
3.4.6 OD value of cell proliferation
The OD value, or optical density value, is a widely used indicator in cellular experiments that measures the amount of light absorbed by the assay. As the number of bacteria in the medium increases, so does the absorbance value within a certain range. Therefore, the OD value is often utilized as a test indicator to assess the proliferation of cells following administration.
A total of seven studies were included to compare the cell proliferation OD between control and experimental groups, with heterogeneity between trials (Tau2 = 0.02, Chi2 = 3062.23, df = 6, I2 = 100%, p < 0.00001), so a random effects model was used. The results showed a statistically significant difference between the treatment and control groups (Z = 11.72, p < 0.00001) with a 95% confidence interval of [MD = −0.63, 95% CI (−0.74, −0.53)]. The results of this study showed a decrease in cell proliferation OD. The results were shown in Figure 9.
3.4.7 Bcl-2 expression level
Bcl-2, also known as the B cell lymphoma/leukemia-2, is an oncogene that plays a crucial role in inhibiting cell apoptosis (Ebrahim et al., 2016). As a major target molecule in the study of molecular mechanisms of apoptosis (Bruckheimer et al., 1998), it has garnered significant attention in antitumor research.
A total of three studies were included to compare the anti-cycloguanine peptides between the control and experimental groups. There was some heterogeneity between the trials (Tau2 = 0.00, Chi2 = 6.50, df = 2, I2 = 69%, p = 0.04), so a random effects model was used. The results revealed a statistically significant difference between the treatment and control groups (Z = 22.33, p < 0.00001) with a 95% confidence interval of [MD = −0.66, 95% CI (−0.71, −0.60)]. The results of this study showed that aconitine reduced the expression of Bcl-2. The results were shown in Figure 10.
Overall, aconitine demonstrated a more significant anti-tumor effect by reducing tumor weight, inhibiting proliferation and migration of tumor cells, promoting apoptosis, and controlling gene/protein expression of cancer cells, including Bcl-2, Bax, Caspase-3, Caspase-8, Cleaved Caspase-9, and others.
When the value of I2 exceeds 50%, it is necessary to conduct a subgroup analysis to investigate the underlying cause of the high heterogeneity. However, the meta-analysis indexes mentioned above have insufficient literature included, such as cell proliferation, thymus index, and number of metastatic lesions indexes. As a result, these indexes may not provide sufficient evidence to support the effectiveness of aconitine against tumors. After reviewing the literature, it became evident that the lack of inclusion of certain studies was because they did not consider the co-administration of aconitine during the screening process.
For instance, one research of Li et al. on the synergistic induction of apoptosis in cervical cancer through the use of quercetin and aconitine, where HeLa cell proliferation was utilized as an outcome indicator (Li et al., 2018); In their study on anti-breast cancer MDA-MB-231BO cells, Guo et al. utilized a combination of osteopontin and aconitine to effectively inhibit cancer cell invasion (Guo et al., 2011); Similarly, Yao et al. found success in treating hepatocellular carcinoma by combining aconitine with Crude Monkshood Polysaccharide, as evidenced by positive experimental outcome indicators such as thymic index (Yao et al., 2021).
The above experiments showed that the meta-analysis indexes used in this study were able to reflect the anti-tumor efficacy of aconitine to some extent. However, due to the absence of quantitative data analysis for integrated and multidimensional purposes in many studies, there is a need for larger sample sizes in in vivo or in vitro experiments to fully understand the multifaceted antitumor effects of aconitine. This is necessary for a rational and proper assessment of its antitumor efficacy.
4 Discussion
4.1 Limitation
When conducting a systematic and comprehensive meta-analysis to evaluate the antitumor efficacy of aconitine, it is important to acknowledge certain limitations. First of all, this study only included literature in Chinese and English, which may introduce a selection bias as there may be relevant studies published in other languages that were not considered. Secondly, the various articles investigating the action of aconitine on tumor cells utilize different methods of administration, control groups, doses, and durations of action. As a result, discrepancies arise in the assays and the results become incomparable. Thirdly, a quality assessment score below 5 indicates a low quality of the methods utilized in the study. Furthermore, many of the studies lacked proper reporting and had flaws in their random assignment and blinding of results. These issues make it challenging to assess the studies’ quality and the trustworthiness of the data.
In addition, the majority of the articles lacked raw data and only included analytical plots and corresponding p values for comparisons between control groups. This limited our ability to refine the data and analyze the anti-tumor efficacy of aconitine using multiple indicators through meta-analysis. Therefore, it is important to assess and interpret the antitumor effects of aconitine at various levels with rationality. In order to conduct a thorough follow-up protocol on the antitumor efficacy study of aconitine, researchers should consider using a similar or equivalent dose range, frequency, duration of action, and control group during the experimental implementation phase. This will enable them to analyze and study the data more accurately in subsequent in-depth studies.
4.2 Implication
The antitumor effects of Aconitine are a complex process that involves multiple factors that have yet to be fully explained. Currently, the main mechanisms of Aconitine anti-tumor properties have been reported in the literature, with a primary focus on the expression of Bax, Bcl-2, Caspase-3, and other proteins, reactive oxygen species damage and the triggering of apoptosis and autophagy in tumor cells.
The literature included in this study commonly used animal indicators such as tumor weight, tumor volume, and thymus index. Cellular experiments frequently measured cell proliferation inhibition rate, apoptosis rate, and cell count at different time periods. In mechanism studies, apoptosis-related targets such as Bax, cyto. C, and caspase-3/9 expression were upregulated, while Bcl-2, pro-caspase 9, MMP2/9, and VEGF expression were downregulated. Based on the changes observed in the expression of these targets, we have formulated a hypothesis that suggests the primary signaling pathway for aconitine anti-tumor effects is NF-κB, and the primary acting immune organ is the thymus. The relevant mechanisms were shown in Figure 11A. The mechanisms through which aconitine induces apoptosis in tumor cells, as discussed earlier, offer numerous opportunities for future researchers to delve deeper into the properties of aconitine alkaloids. Additionally, these tests are acknowledged as essential in the development and clinical application of proprietary Chinese medicines that contain aconite medicinal plants.
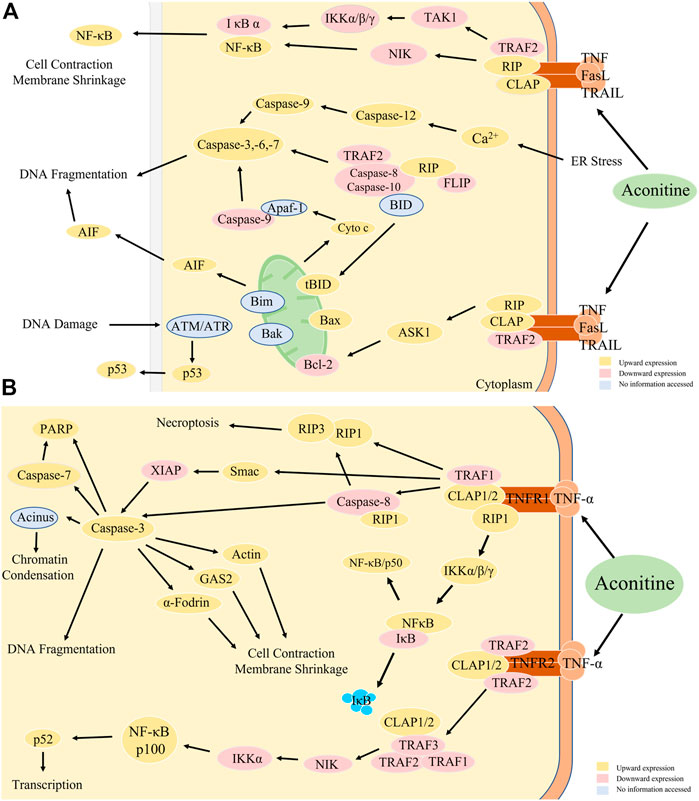
FIGURE 11. (A) Anti-tumor mechanism of aconitine. (B) NF-κB signal path diagram. TRAF2, TNF receptor-associated factor 2; NIK, NF-κB-inducible kinase; TAK1, human transforming growth factor kinase 1. IKK, inhibitor of kappa B kinase, kappa B inhibitory factor kinase; FLIP, FLICE inhibitory protein, apoptosis inhibitory protein; Itch (AIP4), atrophin 1 interacting protein 4; RIP, RNA binding protein; ASK1, apoptosis signal-regulated kinase 1; JNK, c-Jun amino-terminal kinase; AIF, apoptosis-inducing factor; BID, carboxy-terminal fragment; ATM, Ataxia-telangiectasia mutated proteins; ATR, Automatic Target Recognition; p53, human tumor suppressor gene; CLAP, Carbohydrates, Lipid, Nucleic Acid, Protein; IκB, inhibitor of NF-κB; RelA, RELA proto-oncogene, NF-κB subunit; Smac, second mitochondria-derived activator of caspases; GAS2, Recombinant Growth Arrest Specific Protein 2; a-Fodrin, Anti-alpha-cytosolic protein; XIAP, X-linked inhibitor of apoptosis protein; PARP, poly ADP-ribose polymerase.
4.3 Thymus immune organs and T lymphocytes
The thymus, a central immune organ, is situated behind the sternum and in close proximity to the heart. It is divided into two lobes, left and right, and primarily composed of the cortex and the medulla (Rodewald, 2008; Albano et al., 2019). The cortex is mainly composed of larger, immature T lymphocytes, while the medulla contains smaller, less abundant mature T lymphocytes. Due to its significance in the immune system, the thymus is frequently studied in antitumor animal model research.
Cytotoxic T lymphocytes (CTL) are a specialized type of T cell that secretes various immune-related cytokines. Along with natural killer cells, they form a crucial line of defense for the body’s antiviral and antitumor immunity (Rascio et al., 2021). CD8+ T cells and CD4+ T cells are both types of CTL and are believed to have a dominant role in producing an effective anti-tumor response (Kishton et al., 2017).
Cytotoxic T lymphocytes (CTL) are a specialized type of T cell that secretes various immune-related cytokines. Along with natural killer cells, they form a crucial line of defense for the body’s antiviral and antitumor immunity (Rascio et al., 2021). CD8+ T cells and CD4+ T cells are both types of CTL and are believed to have a dominant role in producing an effective anti-tumor response (Kishton et al., 2017).
CD8+ T cells are known to have the ability to directly eliminate tumor cells, making them the preferred immune cells for targeting tumors (Farhood et al., 2019). However, in order to achieve effective anti-tumor immunity, the complementary CD4+ T cells are also necessary (Ahrends et al., 2017).
CD8+ T cells are known to have the ability to directly eliminate tumor cells, making them the preferred immune cells for targeting tumors (Farhood et al., 2019), However, in order to achieve effective anti-tumor immunity, the complementary CD4+ T cells are also necessary (Ahrends et al., 2017). Research has shown that aconitine has multiple effects on cells. It induces apoptosis in tumor cells and activates voltage-dependent Na+ channels in the thymus, which leads to the binding of Ca2+ to downstream molecules and a decrease in free Ca2+ in cells. Additionally, aconitine promotes DP toward SPCD4 and promotes thymic T cell development, maturation, and efflux (Lo et al., 2012). It also increases the percentage of T cells in the spleen and mesenteric lymph nodes, as well as the production of IFN-γ in the spleen and draining lymph nodes, resulting in anti-tumor effects (Zhang, 2021). When aconitine reaches a certain dosage, it can decrease the percentage of Treg obtained by the organism. However, at low doses, not only does it not reduce the percentage of Treg (Cheng et al., 2020), but it can even encourage the differentiation of peripheral blood T cells into Treg (Cheng, 2019). This is done to suppress the function of CD8+ T cells, which ultimately leads to a lower thymic index. Immune cells produce cytokines TNF-α, IL-1β, and IFN-γ, which play crucial roles in immunomodulation and intercellular communication within the immune system. Additionally, these cytokines are essential for the differentiation of memory T cells (Castaneda-Delgado et al., 2017). During tumor development, the body exerts its immune function mainly by regulating the secretion of cytokines (Jiang et al., 2022). Aconitine alkaloids have been shown to promote the secretion of cytokines like TNF-α, IL-1β, and IFN-γ in cancer model mice, thereby enhancing the immune function of the body (Wu et al., 2021).
4.4 Regulatory genes related to autophagy and apoptosis
Autophagy is a cellular process where cells engulf their own cytoplasmic proteins or organelles, encapsulate them into vesicles, and fuse with lysosomes to form autolysosomes. This process allows for the degradation of the encapsulated contents, which satisfies the metabolic needs of the cell and facilitates the renewal of specific organelles. Autophagy is closely linked to cell growth, apoptosis, and internal environmental homeostasis (Ferro et al., 2020) (Glick et al., 2010) (Maiuri et al., 2007). Autophagy is a self-degrading system that is conserved across species and plays a crucial role in maintaining cellular homeostasis during periods of stress (Onorati et al., 2018). However, when autophagy becomes dysregulated, it can have significant implications for human health and disease. Research suggests that inhibiting autophagy may be a promising therapy for advanced cancers. It is important to note that autophagy is not a singular process, but rather requires interaction with various signaling pathways. Furthermore, it is closely linked to apoptosis (Chude and Amaravadi, 2017; Li et al., 2017) (Maheswari et al., 2018). Bcl-2 is the anti-apoptotic gene that is most strongly linked to apoptosis, while Bax is a pro-apoptotic gene that opposes Bcl-2 (Korsmeyer et al., 1993; Cory and Adams, 2005). During the apoptosis signaling process, the Bcl-2 and Bax genes can regulate the activity of the Caspase-3 gene, which in turn controls the apoptotic process via the mitochondrial pathway (Yaidikar and Thakur, 2015). Caspase-3 plays a crucial role in the apoptotic process as the major terminal shear enzyme and the primary executor. Upon initiation of apoptosis, Caspase-3 protein is activated, and its activating protein, Active Caspase-3 protein, can specifically shear DNA. This prompts cytoplasmic coagulation and nucleic acid activation, ultimately leading to apoptosis (Kuper et al., 2011). In contrast, the results of several papers included in this study, the upregulation of Bax expression and the downregulation of Bcl-2 expression demonstrate that aconitine can promote the expression of apoptotic genes and cause excessive autophagy in cancer cells, which leads to apoptosis. In contrast to other papers examined in this study, the findings indicated that aconitine could promote the expression of apoptotic genes/proteins and excessive autophagy in cancer cells by upregulating Bax expression and downregulating Bcl-2 expression, ultimately leading to apoptosis.
4.5 NF-κB signal pathway
Generally, the Bax and Bcl-2 genes work together to maintain a balanced state and facilitate a normal apoptotic process (Siddiqui et al., 2015; Warren et al., 2019). The anti-apoptotic effect of cells is initiated through the activation of a positive cascade of amplified signaling pathways, which are primarily controlled by NF-κB mediated by different survival factors via receptor kinases (Sonenshein, 1997; Dolcet et al., 2005).
Since incorporation of literature demonstrates that aconitine could upregulate Bax and downregulates Bcl-2 expression. Additionally, studies by Ren and Jia demonstrate that aconitine can downregulate the expression of NF-κB pathway (Ren and Zeng, 2008; Jia and Zhang, 2011). Based on this information, it is reasonable to speculate that aconitine achieves its antitumor efficacy through NF-κB signaling pathways. A possible mechanism of aconitine action on the NF-κB signaling pathway were illustrated in Figure 11B.
Under non-activating conditions, NF-κB exists in an inactive form within the cell plasma, and upon extracellular stimulation (e.g., viral or bacterial infection, UV irradiation, etc.), the NF-κB signaling pathway begins to activate (Wu and Kral, 2005). This activation occurs when extracellular signaling factors bind to receptors on the cell membrane, initiating a cascade of downstream responses. The receptor protein receives stimulation and activates I κB kinase (IKK) first (Oeckinghaus and Ghosh, 2009). Intracellular NF-κB-I κB complex is phosphorylated by IKK, which allows for the modification and degradation of I κB, resulting in the release of NF-κB dimers (Hacker and Karin, 2006). These free NF-κB dimers enter the nucleus to bind to genes containing NF-κB binding sites and initiate the transcriptional process (Legrand-Poels et al., 1998). Additionally, NF-κB activates the expression of the I κBα gene, and newly synthesized I κBα re-inhibits NF-κB activity (Baeuerle and Henkel, 1994; Smale, 2012). NF-κB plays a crucial role in regulating cellular responses due to its ability to quickly activate as a master transcription factor without the need for new protein synthesis. It acts as the first responder to harmful stimuli within cells. Several studies (Gilmore et al., 2002; Ali and Mann, 2004; Vasudevan et al., 2004; Clarke et al., 2005) have shown that NF-κB inhibits apoptosis through three primary pathways: ① NF-κB plays a role in both self and other cell apoptosis through the regulation of cytokines; ②NF-κB inhibits apoptosis by inducing or upregulating anti-apoptotic genes; and ③NF-κB inhibits apoptosis by inducing TRAF and IAP.
The NF-κB pathway has a number of known activators, including TNF-α (Liu et al., 2021), interleukin cytokines (He and Karin, 2011), chemokines (Zhao et al., 2021), and colony-stimulating factors (Schreck and Baeuerle, 1990). In addition, some anti-inflammatory molecules such as zinc finger protein (Ye et al., 2019; Zhang et al., 2021), HO-1 (Kim et al., 2019; Saha et al., 2020) and molecules related to apoptosis (TRAF-1, IAP1/IAP2, TRAF1/TRAF2) are also regulated by NF-κB (Zusso et al., 2019; Efferth and Oesch, 2021).
The NF-κB pathway’s anti-apoptotic mechanism involves inhibiting the activation of caspase-8, which in turn inhibits downstream caspase-3. This inhibition is achieved by regulating the expression of TRAF1, TRAF2, c-IAP1, and c-IAP2. Inclusion studies indicate that aconitine is likely to promote the expression of the Bax gene and suppress that of the Bcl-2 gene by regulating the NF-κB signaling pathway. Additionally, aconitine regulates the expression of cell cycle proteins, thereby inhibiting tumor cell proliferation and inducing apoptosis. The anti-apoptotic mechanism of NF-κB is to inhibit the activation of caspase-8 (Dohrman et al., 2005; Schneider et al., 2017) and thus downstream caspase-3 (Luo et al., 2019; Sangaran et al., 2021; Sun et al., 2021) by regulating the expression of TRAF1, TRAF2, c-IAP1, and c-IAP2. Inclusion studies suggested that aconitine was likely to promote the expression of the Bax and suppress that of the Bcl-2 by regulating the NF-κB signaling pathway. Additionally, aconitine could regulate the expression of cell cycle proteins, thereby inhibiting tumor cell proliferation and inducing apoptosis.
5 Conclusion
Although aconitine is highly toxic, it has a remarkable anti-tumor effect. Its mechanism of action is complex, but it inhibits tumor cell proliferation and induces apoptosis. Aconitine activates voltage-dependent Na+ channels in the thymus, promoting thymic T cell development, while also inhibiting Bcl-2 gene expression and activating the downstream gene Caspase-3 to promote tumor cell apoptosis through the regulation of the NF-κB signaling pathway.
Studies have shown that aconitine is highly toxic, with lethal doses (LD50) of 0.2702 ± 0.002 mg/kg in mice, respectively (Zhou et al., 1984). Although aconitine is highly toxic, it has a remarkable anti-tumor effect. It inhibits tumor cell proliferation and induces apoptosis with a complex mechanism, which may inhibit tumor cells by activating voltage-dependent Na+ channels in the thymus and promoting thymic T cell development on the one hand. on the other hand, it inhibits Bcl-2 expression and activates the downstream Caspase-3 to promote tumor cell apoptosis by regulating the NF-κB signaling pathway. However, the precise mechanism of aconitine remains unexplored in both preclinical and clinical trials, whether in vivo or in vitro. At the same time, the challenge of regulating the dosage of aconitine in isolation during clinical trials has led to its frequent use as part of a combination drug in multidrug resistance trials for certain drug-resistant medications. But there are limited in vitro and in vivo preclinical and clinical studies on this subject, making it a key area of investigation for advancing the clinical application of aconitine.
Data availability statement
The original contributions presented in the study are included in the article/supplementary material; further inquiries can be directed to the corresponding author.
Author contributions
GX, conceptualization, data curation and writing-original draft. GX and NX, data extraction and interpretation. SW, conceptualization and writing-review and editing; YZ, writing-review and editing and supervision.
Funding
This study was supported by the National Natural Science Foundation of China (82130113).
Conflict of interest
The authors declare that the research was conducted in the absence of any commercial or financial relationships that could be construed as a potential conflict of interest.
Publisher’s note
All claims expressed in this article are solely those of the authors and do not necessarily represent those of their affiliated organizations, or those of the publisher, the editors and the reviewers. Any product that may be evaluated in this article, or claim that may be made by its manufacturer, is not guaranteed or endorsed by the publisher.
References
Ahrends, T., Spanjaard, A., Pilzecker, B., Bąbała, N., Bovens, A., Xiao, Y., et al. (2017). CD4+ T cell help confers a cytotoxic T cell effector program including coinhibitory receptor downregulation and increased tissue invasiveness. Immunity 47 (5), 848–e5. doi:10.1016/j.immuni.2017.10.009
Albano, F., Vecchio, E., Renna, M., Iaccino, E., Mimmi, S., Caiazza, C., et al. (2019). Insights into thymus development and viral thymic infections. Viruses 11 (9), 836. doi:10.3390/v11090836
Ali, S., and Mann, D. A. (2004). Signal transduction via the NF-kappaB pathway: A targeted treatment modality for infection, inflammation and repair. Cell. biochem. Funct. 22 (2), 67–79. doi:10.1002/cbf.1082
Baeuerle, P. A., and Henkel, T. (1994). Function and activation of NF-kappa B in the immune system. Annu. Rev. Immunol. 12, 141–179. doi:10.1146/annurev.iy.12.040194.001041
Baskar, R., Lee, K. A., Yeo, R., and Yeoh, K. W. (2012). Cancer and radiation therapy: Current advances and future directions. Int. J. Med. Sci. 9 (3), 193–199. doi:10.7150/ijms.3635
Bruckheimer, E. M., Cho, S. H., Sarkiss, M., Herrmann, J., and McDonnell, T. J. (1998). The Bcl-2 gene family and apoptosis. Adv. Biochem. Eng. Biotechnol. 62, 75–105. doi:10.1007/BFb0102306
Cai, G. Q. (2022). Effect of aconitine on proliferation, invasion and apoptosis of prostate cancer cells and its mechanism. Chin. J. Gerontology 42 (09), 2263–2266.
Castañeda-Delgado, J. E., Frausto-Lujan, I., González-Curiel, I., Montoya-Rosales, A., Serrano, C. J., Torres-Juarez, F., et al. (2017). Differences in cytokine production during aging and its relationship with antimicrobial peptides production. Immunol. Investig. 46 (1), 48–58. doi:10.1080/08820139.2016.1212873
Chan, Y. T., Wang, N., and Feng, Y. (2021). The toxicology and detoxification of aconitum: Traditional and modern views. Chin. Med. 16 (1), 61. doi:10.1186/s13020-021-00472-9
Chen, H., Tian, B., Fang, X., Bai, J., Ma, Q., Zhang, Y., et al. (2021). Injectable erythrocyte gel loaded with bulleyaconitine A for the treatment of rheumatoid arthritis. ACS Biomater. Sci. Eng. 7 (12), 5706–5716. doi:10.1021/acsbiomaterials.1c01175
Cheng, C. (2019). Intervention of aconitine on Treg in gastric cancer-bearing mice by regulating PGE2/COX2 pathway. Hefei: Anhui University of Chinese Medicine, 65.
Cheng, C., Zeng, Y. L., Wang, J., Wu, Y., and Zang, L. B. (2020). Effect observation of aconitine on the expression of PGE2 in peripheral blood mononuclear cells of normal 615 mice and its regulation on Treg. J. Liaoning Univ. Traditional Chin. Med. 22 (04), 42–46.
Chude, C. I., and Amaravadi, R. K. (2017). Targeting autophagy in cancer: Update on clinical trials and novel inhibitors. Int. J. Mol. Sci. 18 (6). doi:10.3390/ijms18061279
Clarke, P., Debiasi, R. L., Meintzer, S. M., Robinson, B. A., and Tyler, K. L. (2005). Inhibition of NF-kappa B activity and cFLIP expression contribute to viral-induced apoptosis. Apoptosis 10 (3), 513–524. doi:10.1007/s10495-005-1881-4
Cory, S., and Adams, J. M. (2005). Killing cancer cells by flipping the Bcl-2/Bax switch. Cancer Cell. 8 (1), 5–6. doi:10.1016/j.ccr.2005.06.012
Ding, Y., and Lu, Y. W. (2019). [Comprehensive nursing intervention helps improve medication compliance of prostate cancer patients undergoing endocrine therapy]. Zhonghua Nan Ke Xue 25 (5), 434–436.
Ding, Y. X. (2014). Experimental research on aconite alkaloids in inhibiting human gastric cell line SGC-7901 and the synergistic interaction between aconite alkaloids and chemotherapeutic agents. Nanjing: Nanjing University Of Chinese Medicine, 59.
Ding, Y. X., Zou, X., Wu, J., Ge, H. L., and Hu, S. Y. (2013). Effects of aconite alkaloids on apoptosis and cell cycle of human gastric cancer cell line SGC-7901. Mod. Traditional Chin. Med. Materia Medica-World Sci. Technol. 15 (05), 915–919.
Dohrman, A., Kataoka, T., Cuenin, S., Russell, J. Q., Tschopp, J., and Budd, R. C. (2005). Cellular FLIP (long form) regulates CD8+ T cell activation through caspase-8-dependent NF-kappa B activation. J. Immunol. 174 (9), 5270–5278. doi:10.4049/jimmunol.174.9.5270
Dolcet, X., Llobet, D., Pallares, J., and Matias-Guiu, X. (2005). NF-kB in development and progression of human cancer. Virchows Arch. 446 (5), 475–482. doi:10.1007/s00428-005-1264-9
Du, J., Lu, X., Long, Z., Zhang, Z., Zhu, X., Yang, Y., et al. (2013). In vitro and in vivo anticancer activity of aconitine on melanoma cell line B16. Molecules 18 (1), 757–767. doi:10.3390/molecules18010757
Ebrahim, A. S., Sabbagh, H., Liddane, A., Raufi, A., Kandouz, M., and Al-Katib, A. (2016). Hematologic malignancies: Newer strategies to counter the BCL-2 protein. J. Cancer Res. Clin. Oncol. 142 (9), 2013–2022. doi:10.1007/s00432-016-2144-1
Efferth, T., and Oesch, F. (2021). The immunosuppressive activity of artemisinin-type drugs towards inflammatory and autoimmune diseases. Med. Res. Rev. 41 (6), 3023–3061. doi:10.1002/med.21842
Elmore, S. (2007). Apoptosis: A review of programmed cell death. Toxicol. Pathol. 35 (4), 495–516. doi:10.1080/01926230701320337
Fan, Y., Jiang, Y., Liu, J., Kang, Y., Li, R., and Wang, J. (2016). The anti-tumor activity and mechanism of alkaloids from Aconitum szechenyianum Gay. Bioorg. Med. Chem. Lett. 26 (2), 380–387. doi:10.1016/j.bmcl.2015.12.006
Farhood, B., Najafi, M., and Mortezaee, K. (2019). CD8+ cytotoxic T lymphocytes in cancer immunotherapy: A review. J. Cell. Physiol. 234 (6), 8509–8521. doi:10.1002/jcp.27782
Ferro, F., Servais, S., Besson, P., Roger, S., Dumas, J. F., and Brisson, L. (2020). Autophagy and mitophagy in cancer metabolic remodelling. Semin. Cell. Dev. Biol. 98, 129–138. doi:10.1016/j.semcdb.2019.05.029
Gao, X., Hu, J., Zhang, X., Zuo, Y., Wang, Y., and Zhu, S. (2020). Research progress of aconitine toxicity and forensic analysis of aconitine poisoning. Forensic Sci. Res. 5 (1), 25–31. doi:10.1080/20961790.2018.1452346
Gao, Y., Fan, H., Nie, A., Yang, K., Xing, H., Gao, Z., et al. (2022). Aconitine: A review of its pharmacokinetics, pharmacology, toxicology and detoxification. J. Ethnopharmacol. 293, 115270. doi:10.1016/j.jep.2022.115270
Garmanchouk, L. V., Pyaskovskaya, O. N., Yanish, Y. V., Shlyakhovenko, V. A., Dasyukevich, O. I., and Solyanik, G. I. (2005). Influence of aconitine-containing herbal extract BC1 on proliferative and electrokinetic characteristics of endothelial cells. Exp. Oncol. 27 (4), 262–266.
Gilmore, T., Gapuzan, M. E., Kalaitzidis, D., and Starczynowski, D. (2002). Rel/NF-kappa B/I kappa B signal transduction in the generation and treatment of human cancer. Cancer Lett. 181 (1), 1–9. doi:10.1016/s0304-3835(01)00795-9
Glasziou, P., Vandenbroucke, J. P., and Chalmers, I. (2004). Assessing the quality of research. BMJ 328 (7430), 39–41. doi:10.1136/bmj.328.7430.39
Glick, D., Barth, S., and Macleod, K. F. (2010). Autophagy: Cellular and molecular mechanisms. J. Pathol. 221 (1), 3–12. doi:10.1002/path.2697
Guan, X., Chen, S., and Zhao, Y. (2018). The role of RhoC in malignant tumor invasion, metastasis and targeted therapy. Histol. Histopathol. 33 (3), 255–260. doi:10.14670/HH-11-915
Guan, X., Yang, T. H., Zhang, A. L., Su, M. C., Zhao, R. L., Zhang, C. R., et al. (2015). Mesaconitine extracted from radix aconiti lateralis preparata aconite inhibited proliferation and induced apoptosis of human erythroleukemia (K562) cell line. J. Beijing Univ. Traditional Chin. Med. 38 (07), 467–472+2.
Guan, X., Yang, T. H., Zhang, C. R., and Feng, S. (2017). Induction effect of mesaconitine on apoptosis of K562 and K562/DNR cells and related mechanism. Acta Med. Univ. Sci. Technol. Huazhong 46 (03), 281–284.
Guo, B. F., Liu, S., Ye, Y. Y., and Han, X. H. (2011). [Inhibitory effects of osthole, psoralen and aconitine on invasive activities of breast cancer MDA-MB-231BO cell line and the mechanisms]. Zhong Xi Yi Jie He Xue Bao 9 (10), 1110–1117. doi:10.3736/jcim20111012
Häcker, H., and Karin, M. (2006). Regulation and function of IKK and IKK-related kinases. Sci. STKE 2006 (357), re13. doi:10.1126/stke.3572006re13
Hao, W. Q. (2014). Study on the chemical constituents of the alkaloids from the root of aconitum flavum hand.-mazz and its anti-tumor activities. Yinchuan: Ningxia Medical University, 88.
Hawsawi, Y. M., Al-Zahrani, F., Mavromatis, C. H., Baghdadi, M. A., Saggu, S., and Oyouni, A. (2018). Stem cell applications for treatment of cancer and autoimmune diseases: Its promises, obstacles, and future perspectives. Technol. Cancer Res. Treat. 17, 1533033818806910. doi:10.1177/1533033818806910
He, G., and Karin, M. (2011). NF-κB and STAT3 - key players in liver inflammation and cancer. Cell. Res. 21 (1), 159–168. doi:10.1038/cr.2010.183
Jarrett, A. M., Lima, E., Hormuth, D. N., McKenna, M. T., Feng, X., Ekrut, D. A., et al. (2018). Mathematical models of tumor cell proliferation: A review of the literature. Expert Rev. Anticancer Ther. 18 (12), 1271–1286. doi:10.1080/14737140.2018.1527689
Ji, B., Xia, L., Zhou, F., Mao, G., and Xu, L. (2016). Aconitine induces cell apoptosis in human pancreatic cancer via NF-κB signaling pathway. Eur. Rev. Med. Pharmacol. Sci. 20 (23), 4955–4964.
Ji, X. M. (2021). The study of miR-144 suppressing cells proliferation and migration in colorectal cancer by targeting NRAS. Guangzhou: Southern Medical University, 112.
Ji, X., Yang, M., Or, K. H., Yim, W. S., and Zuo, Z. (2019). Tissue accumulations of toxic aconitum alkaloids after short-term and long-term oral administrations of clinically used radix aconiti lateralis preparations in rats. Toxins (Basel) 11 (6). doi:10.3390/toxins11060353
Jia, M., and Zhang, H. (2011). Effect of ampelopsis japonica and aconite alone and their combination on proliferation and apoptosis of human gastric cancer cell SGC-7901. Mod. J. Integr. Traditional Chin. West. Med. 20 (27), 3388–3390.
Jiang, H., Zhang, Y., Zhang, Y., Wang, X., and Meng, X. (2022). An updated meta-analysis based on the preclinical evidence of mechanism of aconitine-induced cardiotoxicity. Front. Pharmacol. 13, 900842. doi:10.3389/fphar.2022.900842
Jin, L., Wang, C., Liu, J., Li, P., Li, J., Cui, X., et al. (2020). Pseudo-ginsengenin DQ ameliorated aconitine-induced arrhythmias by influencing Ca2+ and K+ currents in ventricular myocytes. RSC Adv. 10 (43), 25999–26005. doi:10.1039/d0ra01683g
Kim, H. N., Park, G. H., Park, S. B., Kim, J. D., Eo, H. J., Son, H. J., et al. (2019). Sageretia thea inhibits inflammation through suppression of NF- κ B and MAPK and activation of Nrf2/HO-1 signaling pathways in RAW264.7 cells. Am. J. Chin. Med. 47 (2), 385–403. doi:10.1142/S0192415X19500198
Kishton, R. J., Sukumar, M., and Restifo, N. P. (2017). Metabolic regulation of T cell longevity and function in tumor immunotherapy. Cell. Metab. 26 (1), 94–109. doi:10.1016/j.cmet.2017.06.016
Klein, G., and Vande Woude, W. G. (2008). Advances in cancer research: Centennial volume. Introduction. Adv. Cancer Res. 100, v–vii. doi:10.1016/S0065-230X(08)00008-0
Korsmeyer, S. J., Shutter, J. R., Veis, D. J., Merry, D. E., and Oltvai, Z. N. (1993). Bcl-2/Bax: A rheostat that regulates an anti-oxidant pathway and cell death. Semin. Cancer Biol. 4 (6), 327–332.
Kotel'nikov, V. M. (1989). [Predictive significance of parameters of tumor cell proliferation]. Eksp. Onkol. 11 (5), 3–9.
Kubatka, P., Uramova, S., Kello, M., Kajo, K., Samec, M., Jasek, K., et al. (2019). Anticancer activities of thymus vulgaris L. In experimental breast carcinoma in vivo and in vitro. Int. J. Mol. Sci. 20 (7). doi:10.3390/ijms20071749
Küper, C., Bartels, H., Beck, F. X., and Neuhofer, W. (2011). Cyclooxygenase-2-dependent phosphorylation of the pro-apoptotic protein Bad inhibits tonicity-induced apoptosis in renal medullary cells. Kidney Int. 80 (9), 938–945. doi:10.1038/ki.2011.199
Legrand-Poels, S., Schoonbroodt, S., Matroule, J. Y., and Piette, J. (1998). Nf-kappa B: An important transcription factor in photobiology. J. Photochem Photobiol. B 45 (1), 1–8. doi:10.1016/S1011-1344(98)00118-3
Li, C. Y., Zhou, Z., Xu, T., Wang, N. Y., Tang, C., Tan, X. Y., et al. (2022). Aconitum pendulum and aconitum flavum: A narrative review on traditional uses, phytochemistry, bioactivities and processing methods. J. Ethnopharmacol. 292, 115216. doi:10.1016/j.jep.2022.115216
Li, M., Xie, X., Chen, H., Xiong, Q., Tong, R., Peng, C., et al. (2020). Aconitine induces cardiotoxicity through regulation of calcium signaling pathway in zebrafish embryos and in H9c2 cells. J. Appl. Toxicol. 40 (6), 780–793. doi:10.1002/jat.3943
Li, S., Yu, L., Shi, Q., Liu, Y., Zhang, Y., Wang, S., et al. (2022). An insight into current advances on pharmacology, pharmacokinetics, toxicity and detoxification of aconitine. Biomed. Pharmacother. 151, 113115. doi:10.1016/j.biopha.2022.113115
Li, X. M., Liu, J., Pan, F. F., Shi, D. D., Wen, Z. G., and Yang, P. L. (2018). Quercetin and aconitine synergistically induces the human cervical carcinoma HeLa cell apoptosis via endoplasmic reticulum (ER) stress pathway. PLoS One 13 (1), e0191062. doi:10.1371/journal.pone.0191062
Li, Y. J., Lei, Y. H., Yao, N., Wang, C. R., Hu, N., Ye, W. C., et al. (2017). Autophagy and multidrug resistance in cancer. Chin. J. Cancer 36 (1), 52. doi:10.1186/s40880-017-0219-2
Lin, C., Clark, R., Tu, P., Bosworth, H. B., and Zullig, L. L. (2017). Breast cancer oral anti-cancer medication adherence: A systematic review of psychosocial motivators and barriers. Breast Cancer Res. Treat. 165 (2), 247–260. doi:10.1007/s10549-017-4317-2
Liu, B., Yan, X., Hou, Z., Zhang, L., and Zhang, D. (2021). Impact of Bupivacaine on malignant proliferation, apoptosis and autophagy of human colorectal cancer SW480 cells through regulating NF-κB signaling path. Bioengineered 12 (1), 2723–2733. doi:10.1080/21655979.2021.1937911
Liu, Y. (2013). Research on pharmacognosy & alkaloids & acute toxicity & anticancer activity of Kazakh medicine aconitum leucostomum worosch. Wulumuqi: Xinjiang Medical University, 62.
Lo, W., Donermeyer, D. L., and Allen, P. M. (2012). A voltage-gated sodium channel is essential for the positive selection of CD4(+) T cells. Nat. Immunol. 13 (9), 880–887. doi:10.1038/ni.2379
Luan, S., Gao, Y., Liang, X., Zhang, L., Wu, Q., Hu, Y., et al. (2022). Aconitine linoleate, a natural lipo-diterpenoid alkaloid, stimulates anti-proliferative activity reversing doxorubicin resistance in MCF-7/ADR breast cancer cells as a selective topoisomerase IIα inhibitor. Naunyn Schmiedeb. Arch. Pharmacol. 395 (1), 65–76. doi:10.1007/s00210-021-02172-5
Luo, R., Chen, X., Ma, H., Yao, C., Liu, M., Tao, J., et al. (2019). Myocardial caspase-3 and NF-κB activation promotes calpain-induced septic apoptosis: The role of Akt/eNOS/NO pathway. Life Sci. 222, 195–202. doi:10.1016/j.lfs.2019.02.048
Ma, F. M., and Yu, T. Q. (2018). Effects of benzoylmesaconin on proliferation and apoptosis of human multipe myeloma cell RPMI-8226. J. Jinan Univ. Sci. Med. Ed. 39 (06), 481–487.
Ma, L., Gu, R., Tang, L., Chen, Z. E., Di, R., and Long, C. (2015). Important poisonous plants in Tibetan ethnomedicine. Toxins (Basel) 7 (1), 138–155. doi:10.3390/toxins7010138
Ma, X. F. (2017). Aconitine regulates the EMT microenvironment to reduce the lung cancer maligant. Zhengzhou: Henan University, 78.
Maheswari, U., Ghosh, K., and Sadras, S. R. (2018). Licarin A induces cell death by activation of autophagy and apoptosis in non-small cell lung cancer cells. Apoptosis 23 (3-4), 210–225. doi:10.1007/s10495-018-1449-8
Maiuri, M. C., Zalckvar, E., Kimchi, A., and Kroemer, G. (2007). Self-eating and self-killing: Crosstalk between autophagy and apoptosis. Nat. Rev. Mol. Cell. Biol. 8 (9), 741–752. doi:10.1038/nrm2239
Oeckinghaus, A., and Ghosh, S. (2009). The NF-kappaB family of transcription factors and its regulation. Cold Spring Harb. Perspect. Biol. 1 (4), a000034. doi:10.1101/cshperspect.a000034
Onorati, A. V., Dyczynski, M., Ojha, R., and Amaravadi, R. K. (2018). Targeting autophagy in cancer. Cancer 124 (16), 3307–3318. doi:10.1002/cncr.31335
Qi, X., Wang, L., Wang, H., Yang, L., Li, X., and Wang, L. (2018a). Aconitine inhibits the proliferation of hepatocellular carcinoma by inducing apoptosis. Int. J. Clin. Exp. Pathol. 11 (11), 5278–5289.
Qi, X., Wang, L., Wang, H., Yang, L., Li, X., and Wang, L. (2018b). Aconitine inhibits the proliferation of hepatocellular carcinoma by inducing apoptosis. Int. J. Clin. Exp. Pathol. 11 (11), 5278–5289.
Raimundo, L., Calheiros, J., and Saraiva, L. (2021). Exploiting DNA damage repair in precision cancer therapy: BRCA1 as a prime therapeutic target. Cancers (Basel) 13 (14). doi:10.3390/cancers13143438
Rao, Z. L., and Peng, C. (2010). Study on the effect of aconitic alkaloid on ras gene expression and its molecular mechanism of antitumor activity. Mod. Prev. Med. 37 (06), 1098–1100+1103.
Rascio, F., Pontrelli, P., and Grandaliano, G. (2021). Cytotoxic T lymphocytes (CTLs) and kidney transplantation: An overview. Methods Mol. Biol. 2325, 203–213. doi:10.1007/978-1-0716-1507-2_14
Reardon, M. J. (2010). Malignant tumor overview. Methodist Debakey Cardiovasc J. 6 (3), 35–37. doi:10.14797/mdcj-6-3-35
Reinbolt, R. E., Mangini, N., Hill, J. L., Levine, L. B., Dempsey, J. L., Singaravelu, J., et al. (2015). Endocrine therapy in breast cancer: The neoadjuvant, adjuvant, and metastatic approach. Semin. Oncol. Nurs. 31 (2), 146–155. doi:10.1016/j.soncn.2015.02.002
Ren, L. Y., and Zeng, S. P. (2008). Effect of radix aciniti praeparata extract on cell apoptosis in transplanted liver cancer H22. Henan Tradit. Chin. Med. (11), 34–37.
Rodewald, H. R. (2008). Thymus organogenesis. Annu. Rev. Immunol. 26, 355–388. doi:10.1146/annurev.immunol.26.021607.090408
Ru, J., Chen, Z., Deng, M., Li, M. H., and Zhang, S. (2020). Aconitine induces apoptosis and inhibits proliferation of gastric adenocarcinoma cell line MGC803 by down-regulating miR-23a. Chin. J. Ethnomedicine Ethnopharmacy 29 (24), 12–17.
Saha, S., Buttari, B., Panieri, E., Profumo, E., and Saso, L. (2020). An overview of Nrf2 signaling pathway and its role in inflammation. Molecules 25 (22). doi:10.3390/molecules25225474
Sangaran, P. G., Ibrahim, Z. A., Chik, Z., Mohamed, Z., and Ahmadiani, A. (2021). LPS preconditioning attenuates apoptosis mechanism by inhibiting NF-κB and caspase-3 activity: TLR4 pre-activation in the signaling pathway of LPS-induced neuroprotection. Mol. Neurobiol. 58 (5), 2407–2422. doi:10.1007/s12035-020-02227-3
Schneider, A. T., Gautheron, J., Feoktistova, M., Roderburg, C., Loosen, S. H., Roy, S., et al. (2017). RIPK1 suppresses a TRAF2-dependent pathway to liver cancer. Cancer Cell. 31 (1), 94–109. doi:10.1016/j.ccell.2016.11.009
Schreck, R., and Baeuerle, P. A. (1990). NF-kappa B as inducible transcriptional activator of the granulocyte-macrophage colony-stimulating factor gene. Mol. Cell. Biol. 10 (3), 1281–1286. doi:10.1128/mcb.10.3.1281-1286.1990
Sena, E., van der Worp, H. B., Howells, D., and Macleod, M. (2007). How can we improve the pre-clinical development of drugs for stroke? Trends Neurosci. 30 (9), 433–439. doi:10.1016/j.tins.2007.06.009
Shao, X., Han, B., Jiang, X. H., Li, F., He, M., and Liu, F. (2019). Effects of benzoyl aconitine on autophagy and apoptosis of human lung cancer cell line in A549. China Pharm. 30 (20), 2782–2788.
Siddiqui, W. A., Ahad, A., and Ahsan, H. (2015). The mystery of BCL2 family: Bcl-2 proteins and apoptosis: An update. Arch. Toxicol. 89 (3), 289–317. doi:10.1007/s00204-014-1448-7
Smale, S. T. (2012). Dimer-specific regulatory mechanisms within the NF-κB family of transcription factors. Immunol. Rev. 246 (1), 193–204. doi:10.1111/j.1600-065X.2011.01091.x
Sonenshein, G. E. (1997). Rel/NF-kappa B transcription factors and the control of apoptosis. Semin. Cancer Biol. 8 (2), 113–119. doi:10.1006/scbi.1997.0062
Suhail, Y., Cain, M. P., Vanaja, K., Kurywchak, P. A., Levchenko, A., Kalluri, R., et al. (2019). Systems biology of cancer metastasis. Cell. Syst. 9 (2), 109–127. doi:10.1016/j.cels.2019.07.003
Sun, Y., Pang, B., Wang, Y., Xiao, J., and Jiang, D. (2021). Baohuoside I inhibits the proliferation of hepatocellular carcinoma cells via apoptosis signaling and NF-kB pathway. Chem. Biodivers. 18 (6), e2100063. doi:10.1002/cbdv.202100063
Tang, M. X., and Sun, G. Z. (1986). Study on the anti tumor and anti metastasis of aconitine and observation on the treatment of cancer. Beijing J. Traditional Chin. Med. (03), 27–28.
Tommelein, J., De Vlieghere, E., Verset, L., Melsens, E., Leenders, J., Descamps, B., et al. (2018). Radiotherapy-activated cancer-associated fibroblasts promote tumor progression through paracrine IGF1R activation. Cancer Res. 78 (3), 659–670. doi:10.1158/0008-5472.CAN-17-0524
Vasudevan, K. M., Gurumurthy, S., and Rangnekar, V. M. (2004). Suppression of PTEN expression by NF-kappa B prevents apoptosis. Mol. Cell. Biol. 24 (3), 1007–1021. doi:10.1128/MCB.24.3.1007-1021.2004
Wang, C. F., Gerner, P., Schmidt, B., Xu, Z. Z., Nau, C., Wang, S. Y., et al. (2008). Use of bulleyaconitine A as an adjuvant for prolonged cutaneous analgesia in the rat. Anesth. Analg. 107 (4), 1397–1405. doi:10.1213/ane.0b013e318182401b
Wang, H. (2020). Experimental study on the function of anti hepatoma and immunoregulation of aconite. Shanghai: Naval Medical University, 92.
Wang, H., Liu, Y., Guo, Z., Wu, K., Zhang, Y., Tian, Y., et al. (2022). Aconitine induces cell apoptosis via mitochondria and death receptor signaling pathways in hippocampus cell line. Res. Vet. Sci. 143, 124–133. doi:10.1016/j.rvsc.2022.01.001
Wang, X., Lin, Y., and Zheng, Y. (2020). Antitumor effects of aconitine in A2780 cells via estrogen receptor β‑mediated apoptosis, DNA damage and migration. Mol. Med. Rep. 22 (3), 2318–2328. doi:10.3892/mmr.2020.11322
Wang, Y., Shan, Y., Wang, Y., Fang, Y., Huang, T., Wang, S., et al. (2019). Aconitine inhibits androgen synthesis enzymes by rat immature Leydig cells via down-regulating androgen synthetic enzyme expression in vitro. Chem. Biol. Interact. 312, 108817. doi:10.1016/j.cbi.2019.108817
Warren, C., Wong-Brown, M. W., and Bowden, N. A. (2019). BCL-2 family isoforms in apoptosis and cancer. Cell. Death Dis. 10 (3), 177. doi:10.1038/s41419-019-1407-6
Wu, J. T., and Kral, J. G. (2005). The NF-kappaB/IkappaB signaling system: A molecular target in breast cancer therapy. J. Surg. Res. 123 (1), 158–169. doi:10.1016/j.jss.2004.06.006
Wu, J., Zhu, Y., Guo, Z., Lou, Y., He, S., Guan, Y., et al. (2018). Aconitum alkaloids, the major components of Aconitum species, affect expression of multidrug resistance-associated protein 2 and breast cancer resistance protein by activating the Nrf2-mediated signalling pathway. Phytomedicine 44, 87–97. doi:10.1016/j.phymed.2017.12.007
Wu, X., Yuan, M. M., Xiong, X. L., and Zhong, G. H. (2021). Advances in the study of chemical constituents and pharmacological effects of aconiti kusnezoffii radix. Drug Eval. 18 (24), 1534–1536.
Wuliji, T. G. S., Liu, Z. B., Wang, H. S., Ding, Y. X., and Su, Y. F. (2008). Effect of total alkaloids from kusnezoff monkshood leaves on immune function in mice. Chin. Traditional Herb. Drugs (07), 1064–1065.
Xiong, H. S., Jiang, C., Gao, R., and Chen, L. H. (2018). Regulatory effects and mechanism of aconitine on proliferation, invasion and migration of hepatoma carcinoma cell MHCC97. Chin. J. Immunol. 34 (05), 688–692.
Xu, X. F., Pi, H. F., Yang, J., Zhu, T., Ruan, H. L., Zhang, Y. H., et al. (2008). A preliminary study on the antitumor cell proliferation activity of aconitum sheath stalk in vitro. Her. Med. (08), 918.
Yaidikar, L., and Thakur, S. (2015). Punicalagin attenuated cerebral ischemia-reperfusion insult via inhibition of proinflammatory cytokines, up-regulation of Bcl-2, down-regulation of Bax, and caspase-3. Mol. Cell. Biochem. 402 (1-2), 141–148. doi:10.1007/s11010-014-2321-y
Yang, L., Chen, Y., Zhou, J., Sun, J., Jiang, W., Liu, T., et al. (2021). Aconitine induces mitochondrial energy metabolism dysfunction through inhibition of AMPK signaling and interference with mitochondrial dynamics in SH-SY5Y cells. Toxicol. Lett. 347, 36–44. doi:10.1016/j.toxlet.2021.04.020
Yang, L. H., Lin, L. M., Wang, Z. M., Li, C., and Li, Z. (2016). [Advance on chemical compounds of Tibetan medicinal plants of Aconitum genus]. Zhongguo Zhong Yao Za Zhi 41 (3), 362–376. doi:10.4268/cjcmm20160302
Yang, Q., Nie, S. Q., Weng, X. G., Li, L. F., and Huang, L. Q. (2005). Experiment studies on anti-tumor effect in vivo and in vitro of aconitum carmichaelii debx. And fritillaria thunbergii MiQ. Used singly or matched. Chin. J. Exp. Traditional Med. Formulae (04), 25–28.
Yao, F., Jiang, G. R., Liang, G. Q., Yuan, Q., Zhu, Y., Liu, M., et al. (2021). The antitumor effect of the combination of aconitine and crude monkshood polysaccharide on hepatocellular carcinoma. Pak. J. Pharm. Sci. 34 (3), 971–979.
Ye, Q., Liu, J., and Xie, K. (2019). Zinc finger proteins and regulation of the hallmarks of cancer. Histol. Histopathol. 34 (10), 1097–1109. doi:10.14670/HH-18-121
Zeng, J., Luo, X., Jiang, N., Qing, Y., Xu, X. Y., and Yu, M. Y. (2007). Study on tumor-inhibitory effect of ChuanWu aqueous extract. J. Sichuan Univ. Sci. Ed. (06), 1344–1348.
Zhang, B. Y. (2021). A report on the translation of health without borders: Epidemics in the era of globalization (chapter 5-7). Chongqing: Sichuan International Studies University, 97.
Zhang, L. J., Tang, X. E., Yao, M. Q., Liu, Y., and Zhao, F. C. (2018). Investigation of the toxicity of three alkaloids in Junggar aconitumon H9c2 cells. J. Xinjiang Med. Univ. 41 (11), 1391–1395.
Zhang, L. J., Zhao, S. J., Deng, R. F., and Zhao, F. C. (2020). Studies on A549 cytotoxicity of aconitine alkaloids and its hydrolyzed products in junggar. China Pharm. 23 (02), 252–255.
Zhang, L., Siyiti, M., Zhang, J., Yao, M., and Zhao, F. (2021). Anti‑inflammatory and anti‑rheumatic activities in vitro of alkaloids separated from Aconitum soongoricum Stapf. Exp. Ther. Med. 21 (5), 493. doi:10.3892/etm.2021.9924
Zhang, Q., Chen, X., Luo, Y., Ren, H., and Qiao, T. (2017). Fuzi enhances anti-tumor efficacy of radiotherapy on lung cancer. J. Cancer. 8 (19), 3945–3951. doi:10.7150/jca.22162
Zhang, R. (2021). Aconitine and PolyIC promote T cell development underlying their anti-tumor effect. Lanzhou: Lanzhou University, 77.
Zhang, W., Shu, H., Fang, L., Tang, N., Li, Y., Guo, B., et al. (2020). Cancer inhibition mechanism of lung cancer mouse model based on dye trace method. Saudi J. Biol. Sci. 27 (4), 1155–1162. doi:10.1016/j.sjbs.2019.12.040
Zhang, W., Zhangyuan, G., Wang, F., Jin, K., Shen, H., Zhang, L., et al. (2021). The zinc finger protein Miz1 suppresses liver tumorigenesis by restricting hepatocyte-driven macrophage activation and inflammation. Immunity 54 (6), 1168–e8. doi:10.1016/j.immuni.2021.04.027
Zhang, X. D., and Wu, Y. T. (2011). Study on apoptosis induction by aconite root in SGC-7901 gastric cancer cell. J. Zhejiang Chin. Med. Univ. 35 (05), 665–668.
Zhao, B., Hou, X. D., Li, H., Qi, X. X., Li, G. G., Liu, L. X., et al. (2014). [Comparative study of Coptidis Rhizoma and Aconiti Kusnezoffii Radix on cell differentiation in lewis lung cancer]. Zhongguo Zhong Yao Za Zhi 39 (14), 2732–2738.
Zhao, H., Wu, L., Yan, G., Chen, Y., Zhou, M., Wu, Y., et al. (2021). Inflammation and tumor progression: Signaling pathways and targeted intervention. Signal Transduct. Target. Ther. 6 (1), 263. doi:10.1038/s41392-021-00658-5
Zheng, S. C., Yan, X. Y., Chen, J., Zhao, S. T., and Wen, C. B. (2017). [Anti-inflammatory mechanism analysis of antirheumatic Chinese medicinal herb Aconiti Radix based on protein interaction network]. Zhongguo Zhong Yao Za Zhi 42 (9), 1747–1751. doi:10.19540/j.cnki.cjcmm.20170121.031
Zhou, X. J., Li, Y. H., Tang, X., Lv, Y. B., and Zhou, J. L. (2019). Aconitine inhibits the proliferation and invasion while induces the apoptosis of esophageal cancer EC-1 cells. Chin. Med. Biotechnol. 14 (04), 335–340.
Zhou, Y. P., Liu, W. H., Zeng, G. Y., Chen, D. H., Li, H. Y., and Song, W. L. (1984). The toxicity of aconitine and its analogues and their effects on cardiac contractive function. Acta Pharm. Sin. (09), 641–646.
Zhu, T. (2008). Studies on the antitumor constituents of aconitum vaginatum. Wuhan: Huazhong University of Science and Technology, 79.
Zou, F., Li, L. L., Dai, M., Nie, T., and Liu, H. C. (2021). Study on the mechanism of aconitine inducing apoptosis of osteosarcoma cells through ROS/JNK signal transduction pathway. Jiangxi Med. J. 56 (06), 799–802.
Keywords: aconitine, malignancy, preclinical studies, meta-analysis, bax, NF-κB, bcl-2
Citation: Xiang G, Xing N, Wang S and Zhang Y (2023) Antitumor effects and potential mechanisms of aconitine based on preclinical studies: an updated systematic review and meta-analysis. Front. Pharmacol. 14:1172939. doi: 10.3389/fphar.2023.1172939
Received: 24 February 2023; Accepted: 17 April 2023;
Published: 27 April 2023.
Edited by:
Muhammad Riaz, Shaheed Benazir Bhutto University, PakistanReviewed by:
Yi Li, China Pharmaceutical University, ChinaHua Yang, China Pharmaceutical University, China
Copyright © 2023 Xiang, Xing, Wang and Zhang. This is an open-access article distributed under the terms of the Creative Commons Attribution License (CC BY). The use, distribution or reproduction in other forums is permitted, provided the original author(s) and the copyright owner(s) are credited and that the original publication in this journal is cited, in accordance with accepted academic practice. No use, distribution or reproduction is permitted which does not comply with these terms.
*Correspondence: Shaohui Wang, winter9091@163.com; Yi Zhang, zhangyi@cdutcm.edu.cn